Abstract
Key message
The leaf rust resistance gene in Thatcher wheat derivative 78–1 was mapped to chromosome 1DS with SNP markers and designated as Lr83.
Abstract
‘Thatcher’ wheat near isogenic line RL6149, a putative derivative of Triticum dicoccoides, was previously determined to carry leaf rust resistance gene Lr64 on chromosome arm 6AL and a second gene temporarily named LrX on chromosome arm 1DS. The objective of this study was to map and characterize LrX in a population of recombinant inbred lines (RILs) that segregated for a single gene. Thatcher line 78–1 with LrX was crossed with Thatcher and individual F2 seedlings and F6 RILs were evaluated for leaf rust response. The 208 F2 plants segregated for a single recessive gene and 148 F6 lines for a single gene. The RILs and parents were characterized by genotyping by sequencing (GBS). Six GBS markers and five Kompetitive Allele-Specific PCR (KASP) markers were used to map LrX on the distal region of chromosome arm 1DS. LrX was 1 centiMorgan (cM) proximal to marker K-IWB38437 and 0.4 cM distal to GBS marker 1D_9037138. Line 78–1 was crossed with Thatcher wheat lines with Lr21, Lr42, and Lr60 for allelism tests. LrX mapped 19.49 cM from Lr21 and 11.93 cM from Lr42. In the cross of line 78–1 with the Thatcher line with Lr60, one recombinant in 1,003 F2 plants was found. LrX and Lr60 are at tightly linked loci on the distal region of chromosome arm 1DS. The gene in line 78–1 was designated as Lr83. Cytological examination of RL6149 provided no evidence of transfer of a chromosome segment of an A- or B-genome chromosome to chromosome 1D.
Similar content being viewed by others
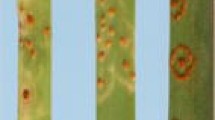
Avoid common mistakes on your manuscript.
Introduction
Leaf rust, caused by Puccinia triticina Eriks., is a widespread and commonly occurring disease of wheat. On a worldwide basis, leaf rust is the leading cause of yield loss in wheat due to disease (Savary et al. 2019). The leaf rust pathogen is highly variable for biotypes or races that differ in ability to infect and cause disease on wheat lines with different leaf rust resistance genes. In the USA, 30–60 races are detected on an annual basis (Kolmer and Fajolu 2022). Currently 82 leaf rust resistance genes (Xu et al. 2022; McIntosh et al. 2022) in wheat have been mapped to chromosome locations and assigned gene designations. However, many of these genes no longer provide resistance, since the P. triticina population is highly dynamic as new virulent races increase rapidly in response to the release of cultivars with effective resistance genes. In the Great Plains region of the USA, cultivars with resistance genes Lr2a, Lr21, Lr24, Lr26, Lr37, and Lr39 have all selected virulent races of P. triticina in the last 30 years (Kolmer 2019).
Given the dynamic nature of P. triticina populations, it is important to characterize germplasm for leaf rust resistance to find new effective resistance genes that can potentially be used in wheat breeding programs to improve leaf rust resistance. The leaf rust resistance gene Lr64 derived from the Thatcher wheat near isogenic line RL6149 was mapped to chromosome 6AL (Kolmer et al. 2019). In the same set of F7 recombinant inbred lines (RILs) that was used to map Lr64, a second unexpected leaf rust resistance gene that mapped to the distal region on chromosome arm 1DS also segregated. Kompetitive Allele-Specific PCR (KASP) markers based on 90 K Infinium iSelect single-nucleotide polymorphic (SNP) markers (Wang et al. 2014) were used to map the 1DS resistance gene. The objective of this study was to further map the 1DS leaf rust resistance gene in a set of RILs that segregated for a single gene with an increased number of markers, to characterize the resistance response with a number of P. triticina isolates, and to cytologically assess the putative wild emmer (Triticum dicoccoides) origin of the gene.
Materials and methods
Phenotyping and inheritance
The F6 line 78–1 from a cross of Thatcher and RL6149 that lacked Lr64, but had a leaf rust resistance gene temporarily designated LrX on chromosome arm 1DS based on seedling infection type and flanking KASP markers (Kolmer et al. 2019), was crossed with Thatcher and F2 seedlings were tested for leaf rust response with P. triticina race isolate BBBDB 1–1, which is avirulent to all of the leaf rust resistance genes in the standard US differential set (Kolmer and Fajolu 2022), except for virulence to the Thatcher wheat line with Lr14a. The F2 seedlings, 78–1, and Thatcher were grown in segmented trays with 48 seedlings per tray, in a greenhouse at 20–23 °C. The seedlings were inoculated when the primary leaves were fully expanded at 7 d with a suspension of urediniospores in Soltrol 170 light mineral oil (Phillips Petroleum, Bartlesville, OK). After inoculation, the plants were dried for 1 h and placed in a dew chamber for 16 h at 18 °C. The seedlings were returned to a greenhouse bench at 20–23 °C with supplemental lighting for a 16 light period and fertilized with a 20–20–20 NPK solution. Infection type (IT) was scored 10 days after inoculation: plants with IT 0 (immune response); (hypersensitive fleck), 1 (small necrotic uredinia), and 2 (small size uredinia with prominent chlorosis) were considered resistant, and plants with IT 3 (moderate size uredinia lacking necrosis or chlorosis) to 4 (large uredinia) were considered susceptible. Plants that had mixed IT were described with the most common IT first. Symbols “–” and “ + ” denoted smaller or larger uredinia, respectively. Two hundred and sixteen F2 plants were advanced to F6 RILs. The F6 RILs and parents were grown in segmented trays with 4–6 seedlings/RIL and were inoculated in two separate tests with isolate BBBDB 1–1 at 7 d when the primary leaves were fully expanded. The F2 and F6 RIL segregation ratios were assessed for conformity to expected Mendelian ratios by Chi-square tests (Steel and Torrie 1980).
Genotyping
The RILs that segregated for response in either test were not considered further for genotyping. The final number of RILs was also reduced to accommodate the genotyping procedure. High quality DNA from 148 RILs and parents was isolated using the method recommended for Diversity Arrays Technology (DArT) (Triticarte Pty Ltd, Canberra, Australia) (Akbari et al. 2006). DNA samples were processed at the University of Minnesota Genomics Center (Minneapolis, MN), using the genotyping-by-sequencing (GBS) (Poland et al. 2012) method. DNA was digested with MspI and PstI to generate 96-plex libraries and ligated with barcode adapters. The DNA libraries were sequenced on one lane of a Novaseq S1 flowcell to generate 100 base pair single end reads (Illumina, San Diego, CA). Only reads that had an average minimum quality score (Q) > 30 were retained. Depth for each RIL exceeded 5 M reads on average.
The filtered GBS reads were aligned with the reference genome Chinese Spring IWGSC RefSeq v2.1 (Zhu et al. 2021) assembly using default parameters in the Burrows-Wheeler Aligner 0.7.5a (Li and Durbin 2009), which was also used to index the reference genome. Variant calling was done using SAMtools 1.6 and BCFtools 1.6 (Li et al. 2009). Alleles were filtered for a minimum read depth of 10, minimum minor allele frequency of 3%, and maximum missing information of 20%. All heterozygous SNPs were converted to ‘H’ and missing alleles to ‘N.’ The SNPs that were H or N for either parent were removed, as were monomorphic SNPs. The SNPs were filtered to obtain sets of markers at 10 or 20% of missing data, respectively.
DNA of the RILs and parents was also genotyped with KASP markers K-IWB38437, K-IWB577, K-IWA713, and K-IWB14612 (Table 1) that were used to map the 1DS leaf rust resistance gene in Kolmer et al. (2019). KASP genotyping followed the standard thermocycler protocol with reagent mixtures of 5 µL described in the LGC Genomics KASP product manual (https://biosearch-cdn.azureedge.net/assetsv6/KASP-genotyping-chemistry-User-guide.pdf). PACE Genotyping Master Mix (3CR Biosciences) was used. Fluorescence was read using a StepOnePlus Real-Time PCR System, and genotypes were determined using the StepOne v2.3 software (Applied Biosystems, Waltham, MA). KASP markers were also developed for the GBS-derived markers 1D_9037138 and 1D_10592628 (Table 1).
Mapping
Selected GBS markers on chromosome arm 1DS and the 1DS KASP markers were combined with the reactions of the F6 RILs to map the segregating leaf rust resistance gene with MapMaker v2.0 for MacIntosh (Lander et al. 1987) using the Kosambi map function with logarithm of odds (LOD) of 10 and r of 0.3. Map order was confirmed with R/qtl (Broman et al. 2003).
Allelism tests
Line 78–1 was crossed with the Thatcher near isogenic lines with Lr21 (RL6043) (McIntosh et al. 1995), Lr60 (RL6172) (Hiebert et al. 2008), and Lr42 (Thatcher*4/WGRC11) (Cox et al. 1994; J. A. Kolmer, unpublished data), and F2 seedlings were inoculated with isolate BBBDB 1–1. Infection types were evaluated 10 d after inoculation. Seedlings that had higher IT than either parent were selected and grown out to F3 lines. Twenty-five seedlings of each F3 line were inoculated with isolate BBBDB 1–1 at 7 d after planting and evaluated for IT at 10 d post-inoculation. Lines that segregated for plants that had low and high IT were considered as being derived from F2 plants heterozygous for leaf rust resistance gene(s), and lines that had plants that were all either resistant or susceptible were considered as being derived from F2 plants that were either homozygous for a resistance gene, segregating for both genes in repulsion, or lacked both resistance gene(s).
Recombination frequency (RF) between linked leaf rust resistance genes was calculated based on 2 times the square root of the frequency of susceptible F2 plants (fs), (RF = 2√fs) (Kuspira and Bhambhani 1984). Genotypes of F2 plants were confirmed in F3 progeny tests. The recombination frequency was converted to map distance using the Kosambi mapping function (Kosambi 1943). Thatcher, line 78–1, and the Thatcher lines with Lr21, Lr42, and Lr60 were inoculated with nine P. triticina isolates that represented different races. All race designations were based on the five letter code used to describe virulence phenotypes in US populations of P. triticina (Kolmer and Hughes 2017). Thatcher lines with Lr21, Lr42, and Lr60 were genotyped with KASP markers developed from GBS markers 1D_9037138 and 1D_10592628, and KASP markers K-IWB38437, K-IWB577, K-IWA713, and K-IWB1461.
Cytology
Mitotic metaphase chromosome preparations from root tips of RL6149 (PBI accession C96.15) and Thatcher followed Lang et al. (2018). Fluorescence in situ hybridization (FISH) and genomic in situ hybridization (GISH) procedures were performed as described in Li et al. (2020). For FISH, oligo probes Oligo-pSc119.2 and Oligo-pTa535 were 5′-end labeled with 6-carboxyfluorescein (6-FAM) and 6-carboxytetramethylrhodamine (Tamra) (Merck, Bayswater, Australia) producing green and red signals, respectively. Chromosomes were counterstained with 4′,6-diamidino-2-phenylindole (DAPI) (Invitrogen Life Science, Carlsbad, CA, USA) and pseudo-colored blue. FISH enabled identification of individual chromosomes. For GISH, total genomic DNA from T. urartu (genome AA) and Aegilops tauschii (genome DD) was labeled with fluorescein-12-dUTP and tetramethyl-rhodamine-5-dUTP (Roche, Basel, Switzerland), which fluoresced green and red, respectively. Total genomic DNA of Ae. speltoides was used as a blocker at a probe-to-blocker ratio of 1:10. GISH enabled distinction of the A-, B-, and D-genome chromosomes and identification of detectable intergenomic translocations if present.
Results
Inheritance and mapping
Individual F2 seedlings of Thatcher × line 78–1 segregated 57 resistant with IT ;22− to 2+, and 151 susceptible with IT 3+ to 4 when tested with isolate BBBDB 1–1, fitting a 1:3 ratio (χ2 = 0.64, p = 0.42) and indicating that 78–1 carries a recessive resistance gene. Over two tests, the F6 RILs segregated 67 resistant and 81 susceptible, fitting an expected segregation of 1:1 (χ2 = 1.31, p = 0.25) and confirming a single gene in 78–1. After alignment of the GBS reads with the reference genome and filtering based on < 20% missingness and minimum read depth of 10, 11 SNPs on chromosome 1D were segregating in the RILs. Six markers that were completely linked with other markers were removed, and a preliminary map based on LrX and the GBS-derived markers 1D_9037138, 1D_10592628, 1D_12018690, 1D_15705818, and 1D_20457400 were constructed. A final linkage map that combined the GBS markers and KASP markers for IWB38437, IWB577, IWA713, and IWB14612 on chromosome arm 1DS (Table 1) and the resistance locus was constructed. LrX mapped to the distal region of chromosome arm 1DS, 1 cM proximal to K-IWB38437 and 0.4 cM distal to 1D_9037138 (Fig. 1). The entire linkage map covered 48.1 cM.
Allelism tests
Leaf rust resistance genes Lr21, Lr42, and Lr60 are also located on chromosome arm 1DS. Tests of allelism were conducted to determine the relationship of LrX with each of these genes. To isolate BBBDB 1–1, line 78–1 had IT ;22− and the Thatcher line with Lr21 (TcLr21) had IT ;22+. From two F2 families of 78–1 × TcLr21, 654 F2 plants had equal or lower IT than the parents, and 43 had IT higher than either parent. When progeny tested, six of the latter plants were classified homozygous susceptible with IT 3+4, a recombination rate of 0.186 and map distance of 19.49 cM between LrX and Lr21. Two of the F3 lines had plants that were all resistant (IT ;1–2−), and 35 lines had plants that segregated for IT ;1–3+.
The Thatcher line with Lr42 had IT ;12− to isolate BBBDB 1–1. From one F2 family of 78–1 × TcLr42, there were 253 plants with equal or lower IT than the parents and 39 with IT higher than either parent. With isolate BBBDB 1–1, progeny tests of the latter 39 plants identified one homozygous susceptible (IT 3+) F3 family, indicating a recombination rate of 0.117 and map distance of 11.93 cM. Sixteen of the F3 lines segregated for plants with IT ranging from ;1 to 22+ and 22 lines had plants with IT ranging from ;1 to 3+.
The Thatcher line with Lr60 had IT ;12+ to isolate BBBDB 1–1. Four F2 families of 78–1 × TcLr60 included 1,003 plants with IT equal to, or lower than, the parents, and 13 had IT higher than either parent to isolate BBBDB 1–1. Twelve of the F3 lines derived from the latter group segregated for IT ;1− to 22+ and one segregated for IT ;22+ and IT 3+. The remaining seeds of this line were planted and tested with isolate BBBDB 1–1. From this line a total of 13 plants had IT ;22+ and 70 plants had IT 3+, approaching a good fit to a 1:3 ratio (χ2 = 3.86, p = 0.049) for segregation of a single recessive gene. Thirty F2 plants with resistant IT were progeny tested with isolate BBBDB 1–1; two lines segregated for plants with IT ;1 and 3+, and the other 28 lines segregated for plants with IT ;1− to ;22+. Although the progeny tests indicated that the genes are not allelic, the genetic distance between them could not be calculated because no homozygous susceptible F2 plants were identified.
KASP haplotypes and resistance phenotyping
The six KASP markers that mapped closest to LrX were used to genotype TcLr21, TcLr42, and TcLr60 (Table 2). Thatcher, TcLr42, and TcLr21 had identical alleles for all six KASP markers; line 78–1 and TcLr60 had the same alleles for all six KASP markers and differed from those for Thatcher. Three resistant and nine susceptible plants from the segregating F3 family from cross 78–1 × TcLr60 were genotyped for the six KASP markers; all 12 plants had the same KASP genotypes as the parents.
The responses of line 78–1, TcLr21, TcLr42, and TcLr60 were also compared using a panel of nine P. triticina races (Table 3). Line 78–1 had intermediate IT of 22+ to all isolates tested, except for isolate MBDSD 8–1 which had a slightly lower IT ;22+. The Thatcher lines with Lr42 and Lr60 had slightly lower IT than 78–1 for all isolates. Isolates TBBGS 95–1 and TNBJS 44–1 had high IT of 3+4 to TcLr21 and low IT to 78–1, TcLr42 and TcLr60.
Cytology
Cytogenetic characterization of RL6149 and Thatcher failed to detect a translocation of an A- or B-genome chromosome segment to chromosome 1D (Fig. 2).
Cytological analysis of chromosomes of Thatcher (a, b) and Thatcher near isogenic line RL6149 (c, d). Sequential FISH (a, c) and GISH (b, d) were performed on the same metaphase cells (a and b, c and d). Chromosomes were hybridized with probes Oligo-pSc119.2–1 labeled with 6-carboxyfluorescein (6-FAM) and Oligo-pTa535-1 labeled with 6-carboxytetramethylrhodamine (Tamra) to generate green and red signals, respectively, enabling identification of individual chromosomes. Chromosomes were counterstained with 4’,6-diamidino-2-phenylindole (DAPI) and pseudo-colored blue. For GISH, total genomic DNA from T. urartu and Aegilops tauschii was labeled with fluorescein-12-dUTP and tetramethyl-rhodamine-5-dUTP, which fluoresced green and red, respectively. Total genomic DNA of Ae. speltoides was used as a blocker, and chromosomes were counterstained with DAPI and pseudo-colored blue. Arrowheads point to chromosome 1D. No A- or B-genome chromatin was detected in chromosome 1D. Bars, 10 μm
Discussion
Segregation of the F6 RILs and mapping data with previously developed KASP markers and new GBS markers confirmed that a single leaf rust resistance gene was present on the distal region of chromosome arm 1DS. This gene conditions an intermediate IT and is recessive in expression. The map order and cM distances of the KASP markers that are in common in the new and previous (Kolmer et al. 2019) maps are very similar. In both maps, the closest KASP marker to the 1DS gene is K-IWB38437, with K-IWB577 13.7 cM proximal to LrX in the current map, and 11.2 cM proximal in the previous map.
Tests of allelism using F2 plants and selected F3 lines derived from crosses of line 78–1 with Thatcher lines possessing Lr21 and Lr42 indicated that LrX was not allelic with these genes. A considerable number of line 78–1 × TcLr42 F2 plants had higher IT than either parent, but only one F3 line was homozygous susceptible. Since the gene in line 78–1 was recessive, most of the F2 plants (German and Kolmer 1992) with higher IT were likely heterozygous and segregated in F3 lines. An increased number of F2 plants would need to be tested to calculate a more accurate map distance between the two genes. Gene Lr42 was mapped to the distal region of 1DS in both hexaploid wheat (Gill et al. 2019) and the diploid donor Ae. tauschii (Lin et al. 2022). In a similar manner, a large number of F3 lines from the line 78–1 × TcLr21 cross also segregated for resistant and susceptible plants, likely caused by F2 plants that were heterozygous for one of the two resistance genes.
Although no F3 line from line 78–1 × TcLr60 was homozygous susceptible, the presence of susceptible plants in one line derived from an F2 plant with higher IT indicated that Lr60 and LrX were not at the same locus. These genes are tightly linked in the distal region of chromosome arm 1DS. The F2 plant from which the F3 line was derived was heterozygous for LrX or Lr60. Since the susceptible plants from this F3 line had KASP genotypes identical to both parents, the plants were derived from the cross and did not originate from pollen contamination from the other parents. The segregation of plants with IT lower than the two parents in F3 lines that were homozygous resistant also indicated that line 78–1 and TcLr60 had different genes. Although over 1,000 F2 plants from 78 to 1 × TcLr60 were tested, more would be needed to increase the probability of obtaining plants that lacked either Lr60 or LrX. Hiebert et al. (2008) estimated that Lr60 was 13.5 cM to distal to Lr21, compared to the 19.49 cM map distance between LrX and Lr21 estimated in the present work. Based on the two mapping studies, LrX is distal to Lr60.
The tetraploid T. dicoccoides line 8404 (Dyck 1994) was the putative source of resistance in RL6149 and therefore should be the source of Lr64 and LrX. Since LrX mapped to chromosome 1DS, the origin of this gene, and presumably also Lr64, is problematic. As indicated by Kolmer (2019), the most likely possibility was that line 8404 was incorrectly identified or was a mixture of tetraploid and hexaploid genotypes, from which a hexaploid individual was the resistant source for RL6149. This is supported by the FISH and GISH analyses (Fig. 2) that provided no evidence of transfer of chromatin from an A- or B-genome chromosome to chromosome 1D, although transfer of a chromosome segment smaller than the level of detection by GISH remains a remote possibility.
LrX conditioned an intermediate IT to isolates of nine races of P. triticina. Wheat lines with LrX singly are predicted to have moderate levels of resistance in field plots based on the seedling IT. Combinations of LrX and other effective leaf rust resistance genes are likely to have lower infection types and improved field resistance. In the F2 plants and F3 lines derived from crosses of line 78–1 with TcLr21, TcLr42, and TcLr60, plants with lower IT than either parent were observed. As noted previously (German and Kolmer 1992; Samborski and Dyck 1982), combinations of effective leaf rust resistance genes, whether adult plant resistance genes only, or genes effective in both seedling and adult plants, usually exhibit lower seedling IT and lower severity levels in field plots. LrX is permanently designated as Lr83. Line 78–1 line has been accessioned in the USDA Small Grains Collection as PI 701,502, small samples of which are available upon request at the USDA-ARS Cereal Disease Laboratory or the USDA National Small Grains Collection.
Data availability
Data will be made available upon reasonable request.
References
Akbari M, Wenzl P, Caig V, Carling J, Yang S, Uszynski G, Mohler V, Lehmensiek A, Kuchel H, Hayden MJ, Howes N, Sharp P, Vaughn P, Rathmell B, Huttner E, Kilian A (2006) Diversity arrays technology (DArT) for high-throughput profiling of the hexaploid wheat genome. Theor Appl Genet 113:1409–1420
Broman KW, Wu H, Sen Ś, Churchill GA (2003) R/qtl: QTL mapping in experimental crosses. Bioinformatics 19:889–890
Cox TS, Raupp WJ, Gill BS (1994) Leaf rust-resistance genes Lr41, Lr42, and Lr43 transferred from Triticum tauschii to common wheat. Crop Sci 34:339–343
Dyck PL (1994) The transfer of leaf rust resistance from Triticum turgidum ssp. dicoccoides to hexaploid wheat. Can J Plant Sci 74:671–673
German SE, Kolmer JA (1992) Effect of gene Lr34 in the enhancement of resistance to leaf rust of wheat. Theor Appl Genet 84:97–105
Gill HS, Li C, Sidhu JS, Liu W, Wilson D, Bai G, Gill BS, Sehgal SK (2019) Fine mapping of the wheat leaf rust resistance gene Lr42. Int J Mol Sci 20:2445
Hiebert CW, Thomas JB, McCallum BD, Somers DJ (2008) Genetic mapping of the wheat leaf rust resistance gene Lr60 (LrW2). Crop Sci 48:1020–1026
Kolmer JA (2019) Virulence of Puccinia triticina, the wheat leaf rust fungus in the United States in 2017. Plant Dis 103:2113–2120
Kolmer JA, Fajolu O (2022) Virulence phenotypes of the wheat leaf rust pathogen, Puccinia triticina, in the United States from 2018 to 2020. Plant Dis 106:1723–1729
Kolmer JA, Hughes ME (2017) Physiologic specialization of Puccinia triticina on wheat in the United States in 2015. Plant Dis 101:1968–1973
Kolmer JA, Bernardo A, Bai G, Hayden MJ, Anderson JA (2019) Thatcher wheat line RL6149 carries Lr64 and a second leaf rust resistance gene on chromosome 1DS. Theor Appl Genet 132:2809–2814
Kosambi DD (1943) The estimation of map distances from recombination values. Ann Eugenics 12:172–175
Kuspira J, Bhambhani RN (1984) A simple method for estimating recombination percentages and linkage intensities from F2 data: examples from Triticum monococcum and other self-fertilizing diploid plant species. Theor Appl Genet 68:61–67
Lander ES, Green P, Abrahamson J, Barlow A, Daly MJ, Lincoln SE, Newburg L (1987) MAPMAKER: an interactive computer package for constructing primary genetic linkage maps of experimental and natural populations. Genomics 1:174–181
Lang T, La SX, Li B, Yu ZH, Chen QH, Li JB, Yang EN, Li GR, Yang ZJ (2018) Precise identification of wheat-Thinopyrum intermedium translocation chromosomes carrying resistance to wheat stripe rust in line Z4 and its derived progenies. Genome 61:177–185
Li H, Durbin R (2009) Fast and accurate short read alignment with Burrows-Wheeler transform. Bioinformatics 25:1754–1760
Li H, Handsaker B, Wysoker A, Fennell T, Ruan J, Homer N, Marth G, Abecasis G, Durbin R (2009) The sequence alignment/map format and SAMtools. Bioinformatics 25:2078–2079
Li J, Dundas I, Dong C, Li G, Trethowan R, Yang Z, Hoxha S, Zhang P (2020) Identification and characterization of a new stripe rust resistance gene Yr83 on rye chromosome 6R in wheat. Theor Appl Genet 133:1095–1107
Lin G, Chen H, Tian B, Sehgal SK, Singh L, Xie J, Rawat N, Juliana P, Singh N, Shrestha S, Wilson DL, Shult H, Lee H, Schoen AW, Tiwari VK, Singh RP, Guttieri MJ, Trick HN, Poland J, Bowden RL, Bai G, Gill B, Liu S (2022) Cloning of the broadly effective wheat leaf rust resistance gene Lr42 transferred from Aegilops tauschii. Nat Commun 13:3044
McIntosh RA, Wellings CR, Park RF (1995) Wheat rusts: an Altas of resistance genes. Kluwer Academic Publishers, Dordrecht, CSIRO Australia
McIntosh, RA, Dubcovsky, J, Rogers WJ, Xia XC, and Raupp, WJ (2022) Catalogue of Gene Symbols for Wheat: 2022 Supplement. Annual Wheat Newsletter Vol 68. https://wheat.pw.usda.gov/ggpages/awn/68/AWN_VOL_68.pdf
Poland JA, Brown PJ, Sorrells ME, Jannink JL (2012) Development of high-density genetic maps for barley and wheat using a novel two-enzyme genotyping-by-sequencing approach. PLoS ONE 7:e32253
Samborski DJ, Dyck PL (1982) Enhancement of resistance to Puccinia recondita by interactions of resistance genes in wheat. Can J Plant Pathol 4:152–156
Savary S, Willocquet L, Pethybridge SJ, Esker P, McRoberts N, Nelson A (2019) The global burden of pathogens and pests on major food crops. Nat Ecol Evol 3:340–439
Steel RGD, Torrie JH (1980) Principles and procedures of statistics: a biometrical approach. McGraw Hill, New York, NY
Wang S, Wong D, Forrest K, Allen A, Chao S, Huang BE, Maccaferri M, Salvi S, Milner SG, Cattivelli L, Mastrangelo AM, Whan A, Stephen S, Barker G, Wieseke R, Plieske J, Lillemo M, Mather D, Appels R, Dolferus R, Brown-Guedira G, Korol A, Akhunova AR, Feuillet C, Salse J, Morgante M, Pozniak C, Luo M-C, Dvorak J, Morell M, Dubcovsky J, Ganal M, Tuberosa R, Lawley C, Mikoulitch I, Cavanagh C, Edwards KJ, Hayden M, Akhunov E (2014) Characterization of polyploid wheat genomic diversity using a high-density 90,000 single nucleotide polymorphism array. Plant Biotechnol J 12:787–796
Xu X, Kolmer J, Li G, Tan C, Carver BF, Bian R, Bernardo A, Bai G (2022) Identification and characterization of the novel leaf rust resistance gene Lr81 in wheat. Theor Appl Genet 135:2725–2734
Zhu T, Wang L, Rimbert H, Rodriguez JC, Deal KR, De Oliveira R, Choulet F, Keeble-Gagnère G, Tibbits J, Rogers J, Eversole K, Appels R, Gu YQ, Mascher M, Dvorak J, Luo MC (2021) Optical maps refine the bread wheat Triticum aestivum cv. Chin Spring Genome Assem Plant J 107:303–314
Acknowledgements
We thank K. Xiao and K. Ristinen for excellent technical assistance and R. McIntosh for comments on the manuscript.
Author information
Authors and Affiliations
Contributions
JAK designed the study, developed the mapping population, phenotyped the population, mapped the population, and prepared the manuscript. PB carried out allele calling using the GBS reads, filtered the markers, and revised the manuscript. JL and PZ performed the cytological analyses and revised the manuscript. MNR designed the KASP markers, conducted the KASP assays and revised the manuscript.
Corresponding author
Ethics declarations
Conflict of interest
On behalf of all authors, the corresponding author states that there is no conflict of interest.
Additional information
Communicated by Evans Lagudah.
Publisher's Note
Springer Nature remains neutral with regard to jurisdictional claims in published maps and institutional affiliations.
Rights and permissions
About this article
Cite this article
Kolmer, J.A., Bajgain, P., Rouse, M.N. et al. Mapping and characterization of the recessive leaf rust resistance gene Lr83 on wheat chromosome arm 1DS. Theor Appl Genet 136, 115 (2023). https://doi.org/10.1007/s00122-023-04361-7
Received:
Accepted:
Published:
DOI: https://doi.org/10.1007/s00122-023-04361-7