Abstract
Hybrid double-skin tubular column (DSTC) with steel inner and fiber-reinforced polymer (FRP) outer tubes belong to a new generation of optimized columns in which concrete is sandwiched between the two tubes. The confinement effect of the sandwich configuration potentially provides additional benefits enhancing the structural behavior of the concrete infill. General guidance on cross-sectional shape sensitivity for nonlinear load carrying capacity is scantly available in literature and standard design specifications. The main objective of present study is to experimentally examine influence of hybrid DSTC cross-sectional shape on its axial-load deflection characteristics when the column undergoes from elastic to ultimate failure states with a final failure by hoop-direction rupture of FRP tube. A total of six-stub hybrid DSTC specimens were tested under axial compression and the observed load-deflection responses were studied for three different cross-sectional shapes of inner and outer tubes, i.e., circle-circle (CC), square-circle (SC), and square-square (SS) specimens. Compared to average peak axial-load capacity of CC specimens (i.e., 1856 kN), SC, and SS specimens showed a capacity drop of about 33 and 38% in this study, respectively. This reduction in axial-load capacity of SC and SS specimens compared to CC shape can be attributed to reduced level of concrete confinement developed due to square cross-sectional shape of the outer FRP tube. Further, the test results provided experimentally verified peak and ultimate axial-load capacities of multiple-shape DSTCs and their load-deflection characteristics for further numerical parametric studies.
Access provided by Autonomous University of Puebla. Download conference paper PDF
Similar content being viewed by others
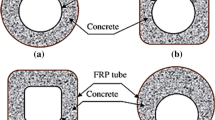
Keywords
- Fiber-reinforced polymer (FRP)
- Hybrid double-skin tubular column (DSTC)
- Peak and ultimate axial-load capacity
- Steel tube local buckling
- Multiple cross-section shapes
1 Introduction
Fiber-reinforced polymer (FRP) when used as a confining material for composite concrete-steel tubular columns, results in a more complex assembly of three constituent materials known as hybrid columns. These FRP-concrete-steel columns provided with inner and outer free surfaces are referred to as double-skin tubular column (DSTC). Hybrid DSTCs have enhanced structural properties due to confined concrete between outer FRP and inner steel tubes, thus provides benefits superior to traditional concrete and steel columns. Because of their structural benefits over traditional columns, such as enhanced load carrying capacity and ductility, reduced weight and better corrosion resistance, etc., hybrid DSTCs have found particular applications for high-rise buildings, industrial structures, and seismic applications. The outer FRP tube with fibers oriented close to the circumferential direction largely provides confinement to sandwiched concrete, whereas inner steel tube also acts as longitudinal reinforcement [1–3]. Typical shape configurations of hybrid DSTC columns based on cross-section shapes of inner and outer tubes is shown in Fig. 1.
Earlier experimental studies have been performed on strengthening of structures using bonded FRP [4, 5]. With further developments in this research field, confinement of concrete with FRP and steel tubes resulted in development of hybrid DSTCs [6]. Recently, DSTCs received significant research attention and emerged with very promising characteristics and structural behavior. Research studies [6–8] demonstrated significant improvement in inelastic deformability and shear resistance of hybrid DSTCs. Other experimental research studies [3, 9–11] were performed to establish axial compressive behavior of hybrid DSTCs for individual cross-sectional shapes such as circle-circle (CC), square-circle (SC), and square-square (SS) shapes. The comparative behavior of DSTCs in particular, the axial load-deflection characteristics (i.e., segments of curves at elastic to peak and ultimate stages) of different cross-sectional shapes have been scantly studied in literature. The axial load-deflection characteristics are expected to depict the hybrid column behavior subjected to different confinements to the sandwiched concrete on account of the shape of the inner and outer tubes.
Against this research necessity, the present study aims to experimentally examine the influence of multiple cross-sectional shapes (i.e., CC, SC, and SS specimens) on axial load-deflection behavior and ultimate axial-load capacity of DSTCs, when column is subjected to compressive loading to undergo from elastic to peak and ultimate states. The present study provides the comparative study on experimentally verified peak and ultimate axial-load capacities and load-deflection characteristics of multiple-shape DSTCs for future parametric studies in this area of research.
2 Experimental Program
2.1 Specimen Details
A total of six specimens of hybrid DSTCs of multiple shapes, i.e., circle-circle (CS), square-circle (SC), and square-square (SS) shapes were prepared and tested under concentric compression. To mitigate experimental uncertainties, two nominally identical specimens were prepared for each column shape. All the FRP tubes irrespective of cross-sectional shape used in this study were originally fabricated by the manufacturer using a filament winding technique with the fibers being at ± 85° to the longitudinal axis of the tubes. The outer FRP tubes used in this study had fixed dimensions, such as diameter or edge length of 208 mm, tube thickness of 4 mm and a height equal to 500 mm. Inner steel tubes were fabricated with 106 mm outer dimension for square tube and 108 mm outer diameter in circular shapes. All steel tubes used had a constant thickness of 2 mm. The total transformed concrete cross-sectional area (i.e., inner steel tube and concrete core) of the resulting DSTC sections was in the ratio of 1:1.31:1.26 for CC, SC, and SS shapes, respectively. The transformed concrete area of CC shape is equal to 27,712 mm2. The space between the outer FRP and inner steel tubes was filled with concrete mix that has 28-days standard cylinder strength equal to 28.13 MPa. A typical label is used in this study to identify test specimens such as “CC-U-I or-II.” In this nomenclature, two shape letters such as CC, SC, and SS are used to represent cross-sectional shape of the DSTC specimens, respectively, as circle-circle, square-circle, and square-square shapes. The letter “U” represented DSTC specimens without any additional steel ribs as stiffeners on inner steel tube. The roman numerical in the label, i.e., “I” or “II” represents two nominally identical specimens for averaging measured test results.
2.2 Material Properties
In accordance with ASTM E8/ E8M-08 [12], six steel-coupons were prepared and tested for tensile strength characteristics. Three-coupons each were obtained from square and circular tubular sections. Upon testing, similar material properties and stress-strain characteristics were observed. Accordingly, average test values obtained for modulus of elasticity (Es), yield stress (fy), and ultimate tensile strength (fu) were 198 GPa, 280 MPa, and 438 MPa, respectively.
Filament-wound glass FRP tubes were supplied by the manufacturer, with uniformity in material parameters across the various shapes of the tubes. All FRP tubes were having fibers inclined at ± 85° to longitudinal axis of the tube. Samples from each supplied FRP tube were tested using split disk test of FRP rings following testing procedures of ASTM D2290-16 [13]. A total of six FRP rings of height 28 mm were prepared and tested on servo controlled universal testing machine type LHC-591. The average values for hoop-direction properties, i.e., tensile strength (fh,frp), elastic modulus (Eh,frp), and rupture strain were obtained as 576 MPa, 40 GPa, and 1.5%, respectively.
The concrete used as infill material between the two tubes was prepared using ordinary Portland cement (OPC) of 43 grade, fine aggregates from river source, and well-graded coarse aggregates of maximum nominal size of 10 mm. A water cement ratio of 0.5 was used in the concrete mix preparation. Standard cubes of size 150 mm × 150 mm × 150 mm were cast and tested in the laboratory for obtaining 28-days average standard cube strength. Average compressive strength of 33.80 MPa was reported from the cube test results, which were performed as per test procedure of BIS. IS: 516-1959 [14].
2.3 Specimen Preparation and Test Setup
For casting of specimens in each column shape (i.e., CC, SC, and SS), a temporary wooden plyboard plane surface was prepared and steel rings of 75 mm height from inner tubes were cut and fixed with this wooden surface. Each of these guiding steel rings were provided with a vertical cut along their height for their easy removal after specimen casting. Inner steel tube was fixed on the outer face of the inner guide ring, and outer tube was placed on the inner side of the outer guide ring fabricated similarly using angle cleats. Such an arrangement ensured concentric placement of the two tubes during specimen castings. Concrete was poured in between the two concentric tubes, compacted properly and water curing were started on the casted specimens after 24 h till 28th day after casting. Finally, the hardened specimens (shown in Fig. 2a) were made ready for testing by smoothening ends of each specimen using light-grinder rubbing to prepare a level surface for uniform load application.
For axial-load deflection measurements, each specimen was provided with a pair of linear-variable displacement transducers (LVDTs) to measure the axial deformations during specimen testing. Finally, all six specimens, two each from CC, SC, and SS shapes, were tested on 300-ton Instron universal testing machine (Fig. 2b). The loading was displacement controlled and applied at a uniform control rate of 0.1 mm per minute. A data logger was connected with both the LVDTs and load-cell to simultaneously record axial-load deflection data along with applied compression loading.
3 Results and Discussion
This experimental study primarily focused on evaluation of ultimate axial-load capacity and load deflection characteristics of stub hybrid DSTCs under axial compression. The shape of the column cross sections (i.e., CC, SC, and SS) was taken as a study parameter. External dimensions of outer tube, i.e., diameter in circular and edge length in square shapes, and tube thickness (4 mm) were kept constant in all specimens. Similarly, inner tube dimensions were nearly equal and thickness was constant (2 mm) in all specimens. The SC and SS specimens had equivalent-concrete area ratios (i.e., ratio of transformed concrete areas of a particular shape to that of CC specimen) of 1.31 and 1.27, respectively. The degree of confinement in a concrete infill can be measured in terms of confinement factor (CF), which is defined as ratio of experimental peak load (\({P}_{p}\)) to sum of individual capacities of steel tube (\({f}_{yi}\) × \({A}_{si}\)) and concrete infill (\({f}_{c}^{^{\prime}}\) × \({A}_{c}\)) of the hybrid columns. Where \({f}_{yi}\) is yield strength and \({A}_{si}\) is cross-sectional area of inner steel tube. \({f}_{c}^{^{\prime}}\) is standard cylinder strength (calculated as 0.8 × cube strength) of concrete, and \({A}_{c}\) is concrete infill area for each column specimen.
Figure 3 shows the experimental peak axial-load capacities (\({P}_{p}\)) of the column specimens observed for each cross-sectional shape. Table 1 summarizes the key experimental results of all tested DSTC specimens. In Table 1, peak axial-load (\({P}_{p}\)) and its corresponding displacement (\({S}_{p}\)) are given. Similarly, the ultimate loads (\({P}_{u}\)) and the corresponding displacements (\({S}_{u}\)) are also noted at the point of FRP rupture. Clearly, CC shape depicts highest peak load (1856 kN) compared to SC (1249 kN) and SS (1155 kN) specimens. The concrete confinement-factors (CF) can be calculated as 2.26 for CC, 1.17 for SC, and 1.11 for SS. The decrease in peak axial-load capacity of SC (33%) and SS (38%) compared to CC shape can be attributed to relative reduction in confinement of concrete infill of about 48% in SC and 51% in SS. Thus, development of concrete confinement is an intrinsic property, which depends on the shape of the column cross section and influences the column peak axial-load capacity.
Figure 4 shows the axial-load displacement characteristics of hybrid CFDSTs. The curves of CC specimens have an approximately ascending bilinear branch followed by a gradual descending branch until the final failure by rupture of outer FRP tubes. In contrast, the curves of SS and SC specimens consisted of a gradually descending branch following an initial linear-ascending portion. It can be seen in Fig. 4c that CC-DSTCs are having a greater effect on the second limb of the response by being larger in extent compared to other two shapes in Figs. 4a, b. Thus, confirming the fact that CC specimens provide a uniform degree of concrete confinement. The descending portion of axial-load displacement curves in Fig. 4 corresponds to the decrease in axial-stiffness of the column on account of reduction in lateral confinement. This decrease in confinement is attributed to local inward buckling of inner steel tube. The CC shape showed reduced and delayed inner tube local-buckling compared to SC and SS shapes, in turn SC has lower inner tube buckling than SS. The ultimate axial-load (\({P}_{u}\)) represented by the last point on axial-load displacement curves in Fig. 4 coincides with FRP tube rupture. The values of \({P}_{u}\) for multiple DSTCs shapes are summarized in Table 1. Average ultimate axial-load (Pu) for CC-shape is observed as maximum (1731 kN) compared to SC (910 kN) and SS (653 kN) shapes. The relative drop in ultimate axial-load compared to CC is 47% for SC and 62% for SS. This reduction in \({P}_{u}\) can be attributed to experimental observation in which inner square tubes showed relatively higher steel tube local bucking compared to circular shape. Moreover, all specimens irrespective of cross-sectional shape finally failed by hoop-direction rupture in FRP tubes, which was seen coinciding with localized failures in concrete and inward local buckling of the inner steel tubes.
4 Summary and Conclusions
This paper presented the test results of an experimental study performed on hybrid-DSTCs. Six DSTC specimens (two each from CC, SC, and SS shapes) were prepared and tested for the ultimate failure load. The specimen preparations and casting, including testing procedures, were provided in detail. The present study focused on influence of cross-sectional shape on the axial compression behavior of hybrid DSTCs, including development of confinement in sandwiched concrete and their peak, and ultimate axial-load capacities. Based on the test results, the following conclusions can be drawn:
-
The ultimate failure of all specimens occurred by hoop-direction rupture of FRP tubes. The FRP tube failure coincided with the localized damage in concrete and corresponding local buckling in inner steel tubes.
-
CC-shaped DSTC specimens exhibited highest peak and ultimate axial loads compared to SC and SS shapes. Relative to CC specimens, the peak and ultimate axial-load capacities in SC shape were reduced by 33 and 47%, respectively. Whereas, the corresponding similar decreases in SS shape were observed as 38 and 62%, respectively.
-
The variation of peak axial-load capacity of DSTC columns with cross-sectional shape can be attributed to the development of varying degree of concrete confinement. Such as CC specimens developed maximum confinement and thus resulted in highest peak load, whereas SS showed the minimum confinement in the present study.
-
The ultimate axial-load capacity of DSTCs, defined at failure of FRP tube is affected by the varying slenderness of inner steel tubes in multiple cross-sectional column shapes. Among, all considered shapes in this study, CC specimens depicted lowest inward local buckling of inner steel tubes, and thus resulted in its highest ultimate axial-load capacity. Whereas, SS showed the lowest ultimate capacity for higher local buckling of inner steel tube.
-
Axial-load deflection characteristics of CC shape showed bilinear ascending curves followed by a mild descending curve depicting evidence of delayed or reduced inner-tube buckling. Whereas, similar test results of SC and SS specimens showed a sharp descending post peak axial load-deflection behavior due to early buckling of inner steel tubes.
The study described herein was performed with rigorous experimental setup and testing procedures and is part of a larger experimental study currently undertaken by the authors for studying behavior of hybrid DSTC columns. The main focus of considering multiple cross-sectional shapes in examining behavior of hybrid DSTCs under axial compression is further extended to stiffened columns [1–3]. Steel flat ribs are provided in stiffened columns on the outer surface of inner steel tube to reduce its local inward buckling. Moreover, additional experimental study is needed to examine column behavior under axial compression by considering a range of DSTC column specimen shapes with different slenderness ratios. Additional parametric studies based on validated finite element models are needed for more general understanding on influence of multiple cross-sectional shapes on concrete confinement and ultimate axial-load capacities of slender hybrid-DSTCs.
References
Zakir M, Sofi FA, Naqash JA (2021) Compressive testing and finite element analysis-based confined concrete model for stiffened square FRP-concrete-steel double-skin tubular columns. J Building Eng 44:103267, ISSN 2352-7102. https://doi.org/10.1016/j.jobe.2021.103267
Zakir M, Sofi FA, Naqash JA (2021) Experimentally verified behavior and confinement model for concrete in circular stiffened FRP-concrete-steel double-skin tubular columns. Structures 33:1144–1157, ISSN 2352-0124. https://doi.org/10.1016/j.istruc.2021.05.010
Zakir M, Sofi FA, Behera S (2021) Nonlinear finite element analysis of circular stiffened FRP-concrete-steel double-skin tubular columns (DSTCs) and experimental compressive behavior of multiple DSTC shapes. Structures 34:3283–3299, ISSN 2352-0124. https://doi.org/10.1016/j.istruc.2021.09.076
Fam A, Rizkalla SH (2001) Behavior of axially loaded concrete-filled circular FRP tubes. ACI Struct J 98(3):280–289
Mirmiran A (2003) Stay-in-place FRP form for concrete columns. Adv Struct Eng 6(3):231–241
Teng JG, Yu T, Wong YL, Dong SL (2007) Hybrid FRP–concrete–steel tubular columns: concept and behavior. Constr Build Mater 21(4):846–854
Teng JG, Lam L (2004) Behavior and modeling of fiber reinforced polymer-confined concrete. J Struct Eng 130(11):1713–1723
Ozbakkaloglu T, Oehlers DJ (2008) Concrete-filled square and rectangular FRP tubes under axial compression. J Compos Constr 12(4):469–477
Peng K, Yu T, Hadi MN, Huang L (2018) Compressive behavior of hybrid double-skin tubular columns with a rib-stiffened steel inner tube. Compos Struct 204:634–644
Zhang B, Teng JG, Yu T (2017) Compressive behavior of double-skin tubular columns with high-strength concrete and a filament-wound FRP tube. J Compos Constr 21(5):1–15 04017029
Fanggi BAL, Ozbakkaloglu T (2015) Square FRP–HSC–steel composite columns: behavior under axial compression. Eng Struct 92:156–171
ASTM E8 / E8M-16ae1 (2016) Standard test methods for tension testing of metallic materials. ASTM International, West Conshohocken, PA
ASTM D2290-04 (2004) Standard test method for apparent hoop tensile strength of plastic or reinforced plastic pipe by split disk method. ASTM International, West Conshohocken, PA
BIS. IS: 516-1959 (2006) Indian standard methods of tests for strength of concrete. Bureau of Indian Standards (BIS), India
Author information
Authors and Affiliations
Corresponding author
Editor information
Editors and Affiliations
Rights and permissions
Copyright information
© 2022 The Author(s), under exclusive license to Springer Nature Singapore Pte Ltd.
About this paper
Cite this paper
Sofi, F.A., Zakir, M., Naqash, J.A. (2022). Experimentally Verified Behavior of Multiple-Shape Hybrid FRP-Concrete-Steel Double-Skin Tubular Columns Under Axial Compression. In: Maity, D., et al. Recent Advances in Computational and Experimental Mechanics, Vol—I. Lecture Notes in Mechanical Engineering. Springer, Singapore. https://doi.org/10.1007/978-981-16-6738-1_14
Download citation
DOI: https://doi.org/10.1007/978-981-16-6738-1_14
Published:
Publisher Name: Springer, Singapore
Print ISBN: 978-981-16-6737-4
Online ISBN: 978-981-16-6738-1
eBook Packages: EngineeringEngineering (R0)