Abstract
The present work aims at characterizing particulate matter (PM) of different size, emitted during biomass gasification in a drop tube furnace (DTF) at 1000 °C. The elemental composition was determined using X-ray fluorescence (XRF) and Electron Paramagnetic Resonance (EPR) analyses. Overall 19 elements were determined and the relative mass concentration of their oxides was identified as macro- (above 3%) and micro-concentration (below 3%). The elements Fe, Mn and S were found in each type of particulates, regardless of the used biomass and gasifying agent. The dominant macro component of char (cyclone particles >10 µm) was Ca (50.56–100% of the total CaO), followed by K, Fe, S, Mn and Cr. Only colza char contained significant portion of P and much lower Fe. The primary macro constituents of PM10–2.5 were Fe, Mn and S. The volatile ash compounds K and Cl are typical constituents of the submicron sized ultrafine particles (UFP), when biomass from agricultural residue was gasified. This confirms the hypothesis that elements, having low boiling point significantly influence UFP formation through the nucleation. Two EPR spectra were obtained for the char samples: a broad signal with g ≈ 2.1–2.6, and a narrow sharp signal with g ≈ 2.002–2.003. The broad EPR signal was attributed to the paramagnetic metal ions Fe3+ and Mn2+, which was in agreement with the XRF analysis. The narrow signal was attributed to the appearance of soot particles.
Access provided by Autonomous University of Puebla. Download chapter PDF
Similar content being viewed by others
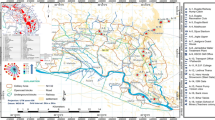
Keywords
1 Introduction
Good understanding of the nature and source of particulate matter (PM) is of crucial importance for developing effective measures for their reduction in the European cities, continuously exceeding the limit values [1].
Currently in Bulgaria, certain attention is drawn on the chemical composition and source apportionment of PM10 and PM2.5, sampled in the atmospheric air. Chuturkova [2] assessed the annual, monthly and maximum concentrations of PM10 and PM2.5 in atmospheric air, within 2007–2014. This investigation involved urbanized and industrial city areas with overall three monitoring stations: city background, transport-oriented, and industrial-oriented. Vlaknenski et al. [3] traced the background air pollution with PM10 in three medium-sized urban areas of Central North Bulgaria during 2007–2010. Veleva et al. [4, 5] measured the daily concentration of PM10 in a period of four seasons in Bulgaria, as well as the chemical composition of the collected samples through Energy Dispersive X-Ray Fluorescent (EDXRF) analysis. The authors identify more than 23 chemical elements in the structure of the collected PM10. Later on, Veleva et al. [6] report the PM10 mass concentration and chemical composition, where more than 20 elements (P, S, Cl, K, Ca, Ti, V, Cr, Mn, Fe, Ni, Cu, Zn Br, Rb, Sr, Y, Zr, Cd, Sn, Sb, I, Ba, Pb) are detected. The PM is sampled during six experimental campaigns in Sofia, within the winter and the summer periods of 2012, 2013 and 2014.
The exceeded PM10 and PM2.5 concentrations in the urban atmosphere is still a challenge for many European Member States, struggling to find efficient solution for various insufficiently controlled processes, such as residential heating and transport [7,8,9]. The role of the local, national and regional PM sources and their specific properties must also be considered. Pateraki et al. [10] chemically characterized PM2.5 and PM1, sampled from Greater Athens Area. The authors extracted 20 different PM-bound polycyclic aromatic hydrocarbons (PAH). Zalakeviciute et al. [11] identified 28 different elements in the structure of PM10, as well as the existence of ions, such as SO42−, NO3− and NH4+. Gvero et al. [12] measured the daily average PM10 concentrations (μg m−3) in Banja Luka city area, with dominant private households, and determined the PM chemical composition. Juda-Rezler et al. [13] studied the PM2.5 for one calendar year and gave the seasonal concentration of PM2.5, 19 trace elements in PM2.5, as well as PM2.5 sources apportionment confirmed the primar role of the following emission sources: residential combustion, exhaust traffic emissions and non-exhaust traffic emissions. Chernishev et al. [14] investigated the particle size distribution and the chemical composition, e.g. the fraction of particles with structured carbon (crystalline phase state) in the sampled PM10, which originated from the exhausts of two-wheeled vehicles that are typically used in the territory of Vladivostok, Russia. According to [14] environmentally persistent free radicals (EPFRs) exist in significant concentration in the atmospheric PM. These EPFRs are primarily emitted from combustion and thermal processing of organic materials, in which the organic combustion by-products interact with transition metal-containing particles to form a free radical-particle pollutant. Vejerano et al. [15] investigated EPFRs in atmospheric PM2.5. The experimentally measured hydroxyl radicals \(( \bullet {\text{OH}})\) were generated from aqueous suspension of ambient PM2.5, using EPR spectrometry and spin trapping. Thus, significant levels of hydroxyl radicals \(( \bullet {\text{OH}})\) were obtained, without the addition of H2O2.
The relation between outdoor and indoor air pollution is investigated in a pilot study of Antova et al. [16]. The authors experimentally measure the concentration of the main pollutants, identified in indoor air, such as: PM10 and PM2.5, ozone, carbon monoxide, carbon dioxide, formaldehyde, nitrogen dioxide, volatile organic compounds, moisture and mold presence in school classrooms. The study confirmed that a large percentage of the schoolchildren in the early school age occupy classrooms where both pollutants—carbon dioxide (90% of the classrooms) and fine particulate matter (50% of the classrooms) significantly exceed the recommended concentrations. The pollutants’ concentration can be directly related to ventilation intensity, classroom occupancy, student activity, heating fuel type and building location.
The typical urban air pollutants affect the respiratory and cardiovascular systems. The release of high levels of PM to the atmosphere greatly concerns the human health and the environment [17]. Therefore, the European legislation [1] sets limit values for particularly harmful substances, such as PM10 and PM2.5 and many others. However, recent surveys show that PM of submicron size is even more dangerous for humans than the monitored PM10 and PM2.5. This type of particulates is often defined as UFP and is related to the anthropogenic emission sources [18]. The toxicity of UFP in air pollution mostly comes from inhalation exposure through the respiratory system, causing significant toxic and health effects. The nose and bronchioles cannot filter out UFP efficiently, thus they have high pulmonary deposition efficiency and could reach the deep inside of the lungs [19]. According to Chen et al. [18] and Cheng [20] the human nose filters <5% of 100-nm particles, but >80% of 1 nm particles during resting breathing. The UFP (PM0.1) generally enter the body through the lungs but translocate to essentially all organs. Compared to fine particles (PM2.5), they cause more pulmonary inflammation and are retained longer in the lungs. Their toxicity is increased with smaller size, larger surface area, adsorbed surface material, and the related physical and chemical characteristics of the particles. Exposure to PM0.1 induces cough and worsens asthma [21, 22], and according to Bhardawaj et al. [23] particles greater than 1 µm aerodynamic diameters generally linger on epithelial.
Biomass is one of the renewable energy sources that can be utilized in different ways, thus serving as valuable feedstock for the chemical industry. It is highly diverse, with different chemical composition, depending on its origin. One way for utilizing biomass is gasification. In order to obtain valuable products, such as chemicals, syngas, etc., biomass is processed in atmosphere with low presence of oxygen [24, 25]. The gasification can be carried out, using different gasifying agents—O2, oxygen-depleted air, CO2, steam or mixtures of these compounds. Usually, moderate to high temperature and pressure, and/or existence of catalyst maximize the yield of the desired products, like CO and H2 [26].
Of scientific, environmental, health and social concern is to study the flue gas, emitted while utilizing concrete biomass types that are annually available in large quantity. Along with the desired valuable products, resulting from the applied utilization process, harmful by-products are emitted. The flue gases of such processes are typical source of pollutants, including PM of different size and chemical composition. Currently, biomass is gaining significant interest on the Bulgarian energy market, but still detailed research is needed about the emission modes and factors of harmful pollutants, like PM with a particular accent on their physical and chemical characteristics.
The present work aims to characterize particulates obtained during biomass gasification in a DTF reactor. In focus were the cyclone particulates with size >10 μm (for simplicity, further in the text they are named char particles) as well as the sampled fine PM and UFP. For that purpose, three types of biomass were gasified, using two different gasifying agents. A particular interest was drawn on the effects of biomass on the PM elemental composition. The elemental composition of the examined particulates was obtained through XRF analysis. In addition, EPR technique was used to study the existence of paramagnetic constituents and the presence of carbon–centered particles in the char. Because this work is still in progress, herein only some preliminary results are presented.
2 Materials and Methods
The selected biomass consists of a woody biomass (softwood and bark) and agriculture residue (colza and sunflower husks (SFH)) that are used for production of solid biofuels in the Member State. The investigated biomass is characterized through proximate, ultimate, ash and calorimetric analyses. The results are presented elsewhere [27].
The gasification installation consists of a biomass feeding system, gas supply system (O2, N2, CO2), vertical DTF reactor, particulate matter sampling system and system for sampling and analyzing the gaseous products. The gasification was carried out at 1000 °C and two different agents—(1) 1% O2 and 99% N2 and (2) 1% O2, 94% N2 and 5% CO2. The biomass feed rate (15 g/h) was constant throughout the entire experiment.
The PM sampling system consists of two cyclones and a Dekati DLPI 13-stage cascade low pressure impactor. The first cyclone was an in-house made in Instituto Superior Técnico, Lisboa, Portugal [25]. The second one was a commercial cyclone, S110 by Dekati®. The role of both cyclones was to capture the particles with diameter larger than 10 µm. The 13-stage cascade (impactor) collects the particulate matter with sizes between 30 nm and 10 μm, with a 50% efficiency cut-off at 13 aerodynamic diameters of PM. The present study examines the char particles and the PM collected on five filters (with D50% = 0.265 μm (PM0.265), D50% = 0.65 μm (PM0.65), D50% = 1 μm (PM1), D50% = 2.5 μm (PM2.5) and D50% = 10 μm (PM10)). For simplicity, further in the text, the above described particulates are entitled as UFP (PM0.265, PM0.65, PM1) and PM (PM2.5 and PM10).
Energy dispersive X-ray fluorescence spectrometer EDX-720 was used to determine the elemental composition of the sampled particulates. The XRF analysis was carried out at room temperature with regard to chemical elements with atomic numbers from 11 (sodium) to 92 (uranium).
The EPR spectra were recorded at room temperature on a JEOL JES-FA 100 EPR spectrometer operating at a frequency of 9.5 GHz (X–band), equipped with a standard TE011 cylindrical resonator. The magnetic field was modulated with a frequency of 100 kHz and amplitude of 0.1 mT. The microwave power was 1 mW. The char samples were placed into a quartz tube (3 mm inner diameter) and were fixed in the cavity center.
3 Results and Discussion
3.1 XRF Analysis
The results from the XRF analyses of the flue gas particulates, sampled during biomass gasification, are qualitatively presented in Table 1. It is an overview of the distribution of chemical elements, that were typically identified in the investigated particulates (char, PM and UFP). In general, Fe, Mn and S were present in all studied particulates, whereas P and Ca were found only in the larger particles (char, PM10 and PM2.5).
Figures 1 and 2 present the relative mass concentration (%) of the obtained oxides, which were conditionally separated in two groups: (a) oxides in macro concentration (above 3%—Fig. 1); (b) oxides in micro concentration (below 3%—Fig. 2). Among the investigated particulate matter, the oxides of Fe, K, Mn, Ca, Cl, S, P were in macro concentration, whereas the oxides of Ti, Co, Ni, Pb, Cu, Zn, Se, Hg were in micro concentration. Similar observations are reported by Veleva et al. [6] in atmospheric PM10, using EDXRF technique. The authors observed the following macro elements: P, S, Cl, K, Ca, Ti, V, Fe (ng.m−3), while the micro elements are: Cr, Mn, Ni, Cu, Zn, Br, Rb, Sr, Zr, Ag, Cd, Sn, Sb, I, Ba, Pb (ng.m−3). According to Obernberger et al. [28] the major ash forming elements (Al, Ca, Fe, K, Mg, Na, P, Si, Ti), typically measured in biomass combustion, are responsible for the ash melting, deposit formation and corrosion. The Cl and S are present in high plant concentrations. Both elements are forming submicron sized PM—the UFP, and are well known for causing deposit formation and corrosion. In addition, Cl is known for causing HCl and polychlorinated dibenzo-p-dioxins and/or dibenzofurans, while S—the SOX emissions [28].
In the present work, the effect of biomass elemental and ash composition on the flue gas particulates (of different size) was considered|. According to the ash analysis [27], Ca and K were the dominant ash constituents in all biomass types. Their relative mass distribution in the biomass ash is as follows: CaO is 33.29 wt % (softwood), 20.44 wt % (SFH) and 29.07 wt % (colza); K2O: 15.76 wt % (softwood), 28.78 wt % (SFH) and 17.80 wt % (colza). Expectedly, the major char constituent was CaO (50.56–100% of the total CaO), regardless of the biomass and the gasifying agent, followed by K, Fe, S, Mn and Cr. According to Gao et al. [29], the majority (83.9–97.5%) of Mg and Ca retains in the chars during biomass pyrolysis/combustion. Potassium (K) is present in significant composition in agriculture residue, because it is among the typical plant’s nutrients. It plays significant role for combating plant diseases, as it increases the plants’ resistance to some pathogens; it also increases cell wall thickness; provides greater tissue stiffness and promotes rapid recovery after injury [30]. The overall sunflower and colza growth and yield decrease in case of potassium deficiency in the soil [31, 32]. The sunflower crops are very sensitive to soil K deficiency and the lack of K often results in both low seed yields and oil concentrations. Sunflower plants have higher resistance to drought and salinity stresses when supplied with adequate quantity of K [33]. The soil fertilization also improves the oil quality, in terms of total unsaturated fatty acid and protein in achene of edible sunflower [34].
The ashes of the studied biomass types contained considerable amount of P and Mg. They were found to increase in the following order: P2O5: 5.78 wt % (softwood) <5.88 wt % (SFH) <11.67% (colza); MgO: 7.44 wt % (softwood) <9.36 wt % (colza) <1.95 wt % (SFH) [27]. However, MgO was not detected in the presently observed samples.
The biomass ash analyses [27] show relatively low content of Fe and Mn below 2 wt %. However, Fe2O3 and MnO were within the major constituents in all PM10–0.265, derived during softwood gasification (regardless of the gasifying agent). The primary macro components of PM10–2.5 were Fe, Mn and S. In addition, about 25% of P and 15% of Ca were found in PM2.5 obtained during gasification of softwood and sunflower husks (SFH), in O2/N2 atmosphere.
The UFP (PM1–0.265) were composed merely of K, Cl, Fe, S, and Mn. Similar observations were reported by Gao and Wu [29] in their investigation, focused on the combustion of volatile matter that was produced in situ, during fast pyrolysis of woody biomass. The authors reported strong correlations between the PM1 yield and the content of (Na + K + Cl), (Na + K), and Cl in the volatiles. In their experiment [29], two-stage pyrolysis/combustion system is used, to produce volatiles (in situ from fast pyrolysis of woody biomass at 800–1000 °C). Then, the volatiles are combusted in DTF at 1300 °C to investigate their contribution to the PM1 emissions. The authors found that the majority of Na, K, and Cl (43.5–97.2%) was volatilized during biomass pyrolysis and the consequent combustion of Na−, K−, and Cl-containing volatiles contributed substantially to the emissions of submicron particulates.
The biomass ultimate analysis [27] showed sulfur content of 0.02 wt % in softwood and <0.05 wt % in SFH and colza. According to Obernberger et al. [28], the total sulfur content of biomass usually varies between 0.02 and 1 wt%. Naydenova et al. [27] measured only SO2 in the flue gases, emitted during biomass oxidation under fluidized bed combustion conditions. The detected SO2 concentration was below 20 ppm, except for the samples investigated at fuel-rich conditions. In the present work, SO3 (according to the XRF-analysis) was obtained in all studied particulates as macro component (up to 19.24%), independently of the biomass type and the gasifying agent. Sulfur is reported as macro element also in the atmospheric PM10, analyzed by Veleva et al. [6].
In line with the work of Gao and Wu [29], Cl was found only in the UFP from agriculture biomass. The smaller the UFP size, the larger the percentage of Cl was obtained. The present results correspond also with the findings of Obernberger et al. [28], who stated that the ash, containing elements like K, Na, P and Cl are typically part of the fly ash, whereas elements like Ca, Mg and Si are usually found in the bottom ash.
Although present in minor concentration the elements: Ti (below 1.3%), Zn (below 0.17%) and Cu (below 0.1%) were found in most of the analysed samples (char, PM and UFP). The measured Cr2O3 varied as macro- and micro-component (between 0.29 and 7.83%), regardless of biomass type and gasifying agent. Generally, the distribution of the micro elements in the investigated PM is highly diverse. Veleva et al. [6] reports Ti, Zn, Cr and Cu merely as microelements in their study on atmospheric PM10. Obernberger et al. [28] commented that the group of minor volatile ash elements, such as As, Cd, Hg, Pb, Zn and others play significant role in gaseous (especially in aerosol) emissions, deposit formation and corrosion, as well as in ash utilization/disposal.
3.2 EPR Analysis
Electron Paramagnetic Resonance (EPR) spectroscopy was used for detecting paramagnetic species in the char particles, sampled from both cyclones. Typical EPR spectra/intensity (in arbitrary units, a.u., but on the same relative scale) of investigated samples are presented in Fig. 3. Two contributions due to different paramagnetic species can be discerned in the room temperature spectra: a broad signal (Signal 1) with g ≈ 2.1–2.6, and a narrow sharp signal (Signal 2) with g ≈ 2.002–2.003.
The broad EPR signal was attributed to paramagnetic metal ions such as Fe3+ and Mn2+, that are typical constituents of the biomass mineral composition and the flying ash. The results correspond well with the elemental composition, obtained by the XRF analysis (Table 1). In the small panels of Fig. 3, with asterisks are noticed lines, which were detected due to a hyperfine structure of the Mn2+ ions. The narrow sharp singlet (again visible in the small panels of the Fig. 3) corresponds to the carbon center or to the soot particles appearing as a paramagnetic product of pyrolysis [35, 36]. In previous studies, soot particles were found to be the dominant part of the UFP [25, 26].
In the EPR spectrum of SFH char samples treated with N2/O2/CO2 (Fig. 3c), in the narrow region of 50 mT, an additional EPR line noted with arrows was observed. This signal (g ≈ 2.00 and line-width 7.9 mT) together with the sharp narrow singlet (g = 2.0022) have been observed in a seperate EPR study of diesel soot particles [37]. The authors ascribe both signals to radicals that are strongly and weakly adsorbed on the carbon particles, respectively.
The EPR parameters (g-factor and line-width ΔHpp) are highly sensitive to the surrounding of the paramagnetic particles (Table 2). For instance, the absorbed oxygen may influence the line-width. Thus, the EPR parameters of the recorded spectra varied depending on the used gasifying agents.
The EPR spectrometry is a relative method in which absolute concentration of the paramagnetic ions/radicals was not measured. However, a comparative study of the quantity of carbon particles was made using the peak-to-peak signal amplitude (EPR intensity). For that purpose, the EPR signal with g values 2.002–2.003 was used. All measured char samples had equal mass and the EPR spectra were recorded simultaneously with an external reference standard of Mn2+/MgO. The mean values from three independent measurements were taken. The highest content of carbon was measured in the char, derived from gasification of SFH with the mixture of N2/O2, whereas the lowest concentration was found in the colza char, using the same gasifying agent.
4 Conclusions
The main goal of the present experimental work was to study the distribution of the biomass ash forming elements in PM of different size. The PM samples were obtained from the flue gases during biomass gasification. The elemental composition of overall 19 elements was determined using XRF analysis. The relative mass concentration of the following oxides was reported: Fe2O3, K2O, SO3, MnO, CaO, Cr2O3, TiO2, Co2O3, NiO, PbO, ZnO, P2O5, MoO3, CuO, SeO2, HgO, PtO2, SiO, in macro- (above 3%) and micro-concentration (below 3%).
The oxides of Fe, Mn and S were found in all studied particulates. The dominant char element was Ca, regardless of the used biomass type and gasifying agent. Besides, Fe, K and S were within the major char elements in SFH and softwood, whereas P was dominating the Fe content in colza gasification.
The Fe2O3 was the most abundant macro component along all studied PM10 and PM2.5 samples, followed by MnO and SO3, as well as P2O5 in the case of SFH and softwood gasification in O2/N2.
In the UFP (PM1, PM0.65 and PM0.265) from agriculture residue (colza and SFH), the macro-components were the oxides of Fe, K and the Cl, whereas in the UFP from softwood, the major component was Fe2O3. These results correspond well with previous investigations [28], stating that vapors of volatile compounds can condense or react on the surface of flying ash particles. Thus, the concentration of condensing ash forming elements such as K, S, Cl, Zn, Pb and others increases with decreasing the particle size.
Highly diverse distribution of the micro constituents was found for all investigated particulates, regardless of the choice of biomass and gasification atmosphere.
The determined EPR spectra showed: a broad signal with g ≈ 2.1–2.6, and a narrow sharp signal with g ≈ 2.002–2.003. The broad EPR signal was attributed to the paramagnetic metal ions Fe3+ and Mn2+, whereas the narrow sharp signal—to the carbon-centered radicals (the soot particles), appearing as paramagnetic product. The highest carbon content was observed in the char derived during SFH gasification in N2/O2 atmosphere, and the lowest one was found in colza char, using the same gasifying agent.
References
Directive 2008/50/EC of the European Parliament and of the Council of 21 May 2008 on ambient air quality and cleaner air for Europe. https://eur-lex.europa.eu/legal-content/EN/TXT/PDF/?uri=CELEX:02008L0050-20150918&from=EN. (4 Jan 2020)
Chuturkova, R.: Particulate matter air pollution (PM10 and PM2.5) in urban and industrial areas. J. Sci. Educ. Innov.; Assoc. Sci. Appl. Res. Int. J. 5, 13-32 (2015). ISSN 1314-9784
Vlaknenski, T., Stoychev, P., Chuturkova, R.: Background air pollution by fine particles in urban areas of central northern Bulgaria. Environ. Technol. 1(22), 82–90 (2015)
Veleva, B., Hristova, E., Nikolova, E., Kolarova, M., Valcheva, R.: Seasonal variation of PM10 elemental composition in urban environment. J. Int. Sci. Publ. Ecol. Saf. 8 (2014). ISSN 1314-7234 (Online). http://www.scientific-publications.net
Veleva, B., Hristova, E., Nikolova, E., Kolarova, M., Valcheva, R.: Elemental composition of air particulate (PM10) in Sofia by EDXRF techniques. J. Chem. Technol. Metall. 49(2), 163–169 (2014)
Veleva, B., Hristova, E., Nikolova, E., Kolarova, M., Valcheva, R.: Experimental study on elemental composition of PM10 in Sofia, 2012–2014. Sci. Technol. Nautical Environ. Stud. 2 (2015). http://dx.doi.org/10.13140/RG.2.1.4570.8562
Bulgaria’s Informative Inventory Report 2019 (IIR), Submission under the UNECE Convention on Long-Range Transboundary Air Pollution, Centre on Emission Inventories and Projections (CEIP), Umweltbundesamt, Vienna, Austria (2019)
Nikolaev, A., Konidari, P.: Development and assessment of renewable energy policy scenarios by 2030 for Bulgaria. Renew. Energy 111, 792–802 (2017). https://doi.org/10.1016/j.renene.2017.05.007
Naydenova, I., Petrova, T., Velichkova, R., Simova, I.: PM10 exceedance in Bulgaria. In: CBU International Conference Proceedings, 21–23 Mar 2018, Prague, Czech Republic, vol. 6, pp. 1129–1138 (2018). http://dx.doi.org/10.12955/cbup.v6.1305
Pateraki, St., Asimakopoulos, D.N., Maggos, Th., Assimakopoulos, V.D., Bougiatioti, A., Bairachtari, K., Vasilakos, Ch., Mihalopoulos N.: Chemical characterization, sources and potential health risk of PM2.5 and PM1 pollution across the Greater Athens Area. Chemosphere 241, 125026 (2020)
Zalakeviciutea, R., Rybarczyk, Y., Granda-Albuja, M.G., Suarez, M.V.D., Alexandrino, K.: Chemical characterization of urban PM10 in the tropical andes. Atmos. Pollut. Res. 11, 343–356 (2020)
Gvero, P., Radić, R., Kotur, M., Kardaš, D.: Urban air pollution caused by the emission of PM10 from the small household devices and abatement measures. Therm. Sci. 152–152 (2018). https://doi.org/10.2298/tsci180119152g
Juda-Rezler, K., Reizer, M., Maciejewska, K., Błaszczak, B., Klejnowski, K.: Characterization of atmospheric PM2.5 sources at a Central European urban background site. Sci. Total Environ. 713, 136729 (2020)
Chernyshev, V.V., Zakharenko, A.M., Ugay, S.M., Hien, T.T., Hai, L.H., Kholodov, A.S., Burykina, T.I., Stratidakis, A.K., Mezhuev, Ya. O., Tsatsakis, A.M., Golokhvast, K.S.: Morphologic and chemical composition of particulate matter in motorcycle engine exhaust. Toxicol. Rep. 5, 224–230 (2018)
Vejerano, E.P., Rao, G., Khachatryan, L., Cormier, S.A., Lomnicki, S.: Environmentally persistent free radicals: insights on a new class of pollutants. Environ. Sci. Technol. 52(5), 2468–2481 (2018). https://doi.org/10.1021/acs.est.7b04439
Antova, T., Panev, T.I., Tzoneva, M.Tz., Sidjimov, M.A., Lukanova, R.T.: Indoor air quality assessment (pilot study). J. Int. Sci. Publ. Ecol. Saf. 13, 77–85 (2019). ISSN 1314-7234
Lopes, V.S.B.: Effect of Particle Size on the Burnout and Emissions of Particulate Matter from Biomass Combustion in a Drop Tube Furnace. Instituto Superior Técnico, Universidade de Lisboa, Lisboa, Portugal, May, Thesis to obtain the Master of Science Degree (2016)
Chen, R., Hu, B., Liu, Y., Xu, J., Yang, G., Xu, D., Chen, C.: Beyond PM2.5: The role of ultrafine particles on adverse health effects of air pollution. Biochimica et Biophysica Acta 1860, 2844–2855 (2016). http://dx.doi.org/10.1016/j.bbagen.2016.03.019
Chow, J.C., Watson, J.G.: Overview of ultrafine particles and human health. WIT Trans.Ecol. Environ. 99, (2006). © 2006 WIT Press. https://doi.org/10.2495/rav060601
Cheng, Y.S.: Aerosol deposition in the extrathoracic region. Aerosol Sci. Technol. 37, 659–671 (2003)
Schraufnagel, D.E.: The health effects of ultrafine particles. Exp. Mol. Med. 52, 311–317 (2020). https://doi.org/10.1038/s12276-020-0403-3
Buzea, C., Pacheco, II., Robbie, K.: Nanomaterials and nanoparticles: sources and toxicity. Biointerphases 2, MR17-71 (2007)
Bhardawaj, A., Habib, G., Kumar, A., Singh, S., Nema, A.K.: A review of ultrafine particle-related pollution during vehicular motion, health effects and control. J. Environ. Sci. Public Health 1(4), 268–288 (2017). https://doi.org/10.26502/jesph.96120024
Basu, P.: Biomass Gasification and Pyrolysis: Practical Design and Theory. Elsevier Sci Technol, Burlington, United States (2010)
Duarte, F.S.: Biomass Gasification in a Drop Tube Furnace. Thesis to obtain the Master of Science Degree in Mechanical Engineering, Instituto Superior Técnico, Lisboa (2018)
Adánez-Rubio, I., Ferreira, R., Rio, T., Alzueta, M.U., M. Costa, M.: Soot and char formation in the gasification of pig manure in a drop tube reactor. Fuel 281, 118738 (2020). https://doi.org/10.1016/j.fuel.2020.118738
Naydenova, I., Sandov, O., Wesenauer, F., Laminger, T., Winter, F.: Pollutants formation during single particle combustion of biomass under fluidized bed conditions: an experimental study. Fuel 278, (2020)
Obernberger, I., Brunner, T., Baernthaler, G.: Chemical properties of solid biofuels—significance and impact. Biomass Bioenergy 30, 973–982 (2006). https://doi.org/10.1016/j.biombioe.2006.06.011
Gao, X., Wu, H.: Combustion of volatiles produced in situ from the fast pyrolysis of woody biomass: direct evidence on its substantial contribution to submicrometer particle (PM1) emission. Energy Fuels 25(9), 4172–4181 (2011). https://doi.org/10.1021/ef2008216
Alves, A.N., Guedes de Souza, F., Chaves, L.H.G., Alves de Sousa, J., Feitosa de Vasconcelos, A.C.: Effect of nutrient omission in the development of sunflower BRS-122 in greenhouse conditions. Rev. Fac. Nac. de Agronomía Medellín 72(1), 8663 (2019). http://dx.doi.org/10.15446/rfnam.v72n1.69388
Thrace Agricultural Research Institute, Republic of Turkey, Ministry of Agriculture and Forestry, Effects of Potassium Fertilization on Sunflower (Helianthus annuus L.) and Canola (Brassica napus ssp. oleifera L.) Growth (2020). https://arastirma.tarimorman.gov.tr/ttae/Sayfalar/EN/Detay.aspx?SayfaId=151
Cheema, M.A., Wahid, M.A., Sattar, A., Rasul, F., Saleem, M.F.: Influence of different levels of potassium on growth, yield and quality of canola (Brassica napus L.) cultivars. Pak. J. Agri. Sci. 49(2), 163–168 (2012)
IPNI Canada, Potassium fertilization is critical to sunflower. http://china.ipni.net/article/CNP-3225. Last accessed 23 Aug 2018
Li, S., Duan, Y., Guo, T., Zhang, P., He, P., Majumdare, K.: Sunflower response to potassium fertilization and nutrient requirement estimation. J. Integr. Agric. 17(12), 2802–2812 (2018). https://doi.org/10.1016/S2095-3119(18)62074-X
Yordanov, N.D., Lubenova, S., Sokolova, S.: On the possibility for separate determination of pyrolyzed products (soot and polycyclic aromatic hydrocarbons) in aerosols by EPR spectrometry. Atmos. Environ. 35, 827–831 (2001)
Yamanaka, C., Matsuda, T., Ikeya, M.: Electron spin resonance of particulate soot samples from automobiles to help environmental studies. Appl. Radiat. Isot. 62, 307–311 (2005)
Abi-Aad, E., Cousin, R., Pruvost, C., Courcot, D., Noirot, R., Rigaudeau, C., Aboukaïs, A.: EPR investigation and reactivity of diesel soot activated (or not) with cerium compounds. Top. Catal. 16–17(1–4), 263–268 (2001)
Acknowledgements
The authors would like to acknowledge the financial support from the National Science Program “Environmental Protection and Reduction of Risks of Adverse Events and Natural Disasters”, approved by the Resolution of the Council of Ministers No 577/17.08.2018 and supported by the Ministry of Education and Science of Bulgaria (Agreement No D01-230/06.12.2018 and Agreement No Д01-322/18.12.2019).
Tsvetelina Petrova, Ph.D. expresses her deep gratitude to the Internal Competition of Technical University of Sofia—2020 for the scientific project “Perspective leaders”, Contract № 201ПP0017-10/26.05.2020 “Composition and characteristics of by-products, formed during biofuels utilization”.
Author information
Authors and Affiliations
Corresponding author
Editor information
Editors and Affiliations
Rights and permissions
Copyright information
© 2021 The Author(s), under exclusive license to Springer Nature Switzerland AG
About this chapter
Cite this chapter
Petrova, T., Naydenova, I., Ferreira, R., Karakirova, Y., Costa, M. (2021). Chemical Characteristics of Flue Gas Particulates: An Experimental Investigation. In: Dobrinkova, N., Gadzhev, G. (eds) Environmental Protection and Disaster Risks. EnviroRISK 2020. Studies in Systems, Decision and Control, vol 361. Springer, Cham. https://doi.org/10.1007/978-3-030-70190-1_15
Download citation
DOI: https://doi.org/10.1007/978-3-030-70190-1_15
Published:
Publisher Name: Springer, Cham
Print ISBN: 978-3-030-70189-5
Online ISBN: 978-3-030-70190-1
eBook Packages: EngineeringEngineering (R0)