Abstract
Rodent transgenic parasites are useful tools for the preclinical evaluation of malaria vaccines. Over the last decade, several studies have reported the development of transgenic rodent parasites expressing P. falciparum antigens for the assessment of vaccine-induced immune responses, which traditionally have been limited to in vitro assays. However, the genetic manipulation of rodent Plasmodium species can have detrimental effects on the parasite’s infectivity and development. In this chapter, we present a few guidelines for designing transfection plasmids, which should improve transfection efficiency and facilitate the generation of functional transgenic parasite strains. In addition, we provide a transfection protocol for the development of transgenic P. berghei parasites as well as practical methods to assess the viability and infectivity of these newly generated strains throughout different stages of their life cycle. These techniques should allow researchers to develop novel rodent malaria parasites expressing antigens from human malaria species and to determine whether these transgenic strains are fully infectious and thus represent stringent platforms for the in vivo evaluation of malaria vaccine candidates.
Access provided by CONRICYT – Journals CONACYT. Download protocol PDF
Similar content being viewed by others
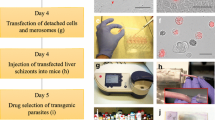
Key words
1 Introduction
Despite significant advances in our understanding of the biology and immunogenicity of Plasmodium parasites, a safe and fully effective vaccine against malaria has not been developed.
Research on malaria vaccine development has traditionally targeted one of the three stages of the Plasmodium parasite life cycle: (1) pre-erythrocytic stages, from sporozoite inoculation through mosquito bites to hepatocyte infection; (2) asexual blood stages, from the release of merozoites within an infected hepatocyte to their continuous infection of red blood cells; and (3) sexual stages, from the development of blood-stage gametocytes in the mammalian host to the development of sporozoites in the mosquito. Among these strategies, pre-erythrocytic vaccines are appealing as they have the potential to prevent infection and clinical disease, as well as block transmission.
The circumsporozoite protein (CSP), the most abundant protein on the surface of Plasmodium sporozoites, is one of the best studied malaria antigens and a leading vaccine candidate. Studies in animal models and human volunteers have shown that antibodies and T-cell responses against CSP can inhibit sporozoite infection in mammalian hosts and provide protective immunity [1, 2]. Human vaccine trials using the CSP-based malaria vaccine candidate RTS,S—currently the most successful subunit malaria vaccine formulation —have shown that RTS,S is capable of preventing the development of clinical malaria in 30–50 % of vaccinees. In addition, the RTS,S vaccine was found to reduce the incidence of severe disease in children living in malaria endemic areas [3, 4]. In spite of these encouraging advances, it is apparent that RTS,S does not confer protection to a large proportion of vaccinees and its protective efficacy seems to wane after 1–2 years [3–5]. These results may, in part, be explained by the over-reliance of RTS,S on a single antigen , CSP. And while CSP remains the most studied target of pre-erythrocytic malaria vaccines, additional antigens capable of eliciting a protective immune response have been identified [6]. It is hoped that more complex subunit vaccines with multiple antigens, including CSP, may greatly improve protective efficacy [7].
Rodent and nonhuman primate malaria parasites have been helpful for addressing basic questions in malaria biology and are valuable models for the preclinical evaluation of vaccine candidates. However, a fundamental limitation of murine malaria species is that rodent orthologues (i.e., functionally similar genes) are antigenically distinct from human-infecting Plasmodium species. This is a critical shortcoming of rodent models as conclusions based on rodent malaria antigens, while valuable from a conceptual view point, are limited as they cannot account for significant differences in the antigenic structure of murine and human parasite antigens. The development of transgenic rodent parasites expressing human malaria antigens has emerged as a practical solution to this problem [8]. Over the last few years newly developed transgenic parasite strains have been used for the preclinical evaluation of vaccine candidates against malaria pre-erythrocytic stages [9–12]. Importantly, these parasite strains have facilitated the systematic evaluation of different immunizing routes, antigen constructs, adjuvant formulations, and immunization regimens. And while the optimization of transfection protocols for rodent Plasmodium species has significantly simplified genetic manipulation [13, 14], the substitution and/or deletion of essential genes or regulatory sequences can negatively impact the parasite’s infectivity and life cycle [10, 15, 16]. This is a major obstacle because transgenic parasite strains with decreased viability and virulence are not adequate tools to evaluate vaccine candidates.
In this chapter, we describe useful guidelines for the design of transfection plasmids, provide a standardized P. berghei transfection protocol, and present practical methodology to assess the viability and infectivity of transgenic P. berghei parasites throughout their life cycle. Importantly, these methods have been applied to transgenic strains developed by our group and others, in which different endogenous genes of P. berghei have been replaced by orthologues found in human malaria Plasmodium species [9, 11, 12]. Ultimately, the careful selection of highly infectious strains is critical for the stringent evaluation of malaria vaccine candidates in vivo.
2 Materials
2.1 Transfection of Rodent Malaria Parasites
2.1.1 Establishment of Infection and Subpassage to Schizont Donor Mice
-
1.
Frozen blood aliquot of wild-type P. berghei parasites.
-
2.
Three Swiss Webster mice (5–8 weeks old; ~25 g) (see Note 1 ).
-
3.
Hanks’ Balanced Salt Solution (HBSS) (Gibco) supplemented with 1 % (v/v) heat-inactivated mouse serum (Sigma).
-
4.
Scissors.
-
5.
Glass slides (25 × 75 mm).
-
6.
Methanol.
-
7.
Giemsa stain (Sigma).
-
8.
100× Giemsa buffer: 59.24 g Na2HPO4, 36.38 g NaH2PO4H2O, 1000 ml deionized water.
-
9.
1 cc Insulin syringe (28G ½).
-
10.
1.5 ml microcentrifuge tubes.
-
11.
1000 μl and 20 μl micropipettes with corresponding plastic tips.
2.1.2 Schizont In Vitro Culture
-
1.
Glass slides (25 × 75 mm).
-
2.
Methanol.
-
3.
Giemsa stain (Sigma).
-
4.
100× Giemsa buffer: 59.24 g Na2HPO4, 36.38 g NaH2PO4H2O, 1000 ml deionized water.
-
5.
Heparin solution (200 U/ml in 1× PBS).
-
6.
Complete culture medium (200 ml per culture): RPMI 1640 medium with l-glutamine (Gibco), 25 mM Hepes buffer, 5 U/ml penicillin, 5 mg/ml streptomycin, adjust pH to 7.3 and filter (0.22 μm pore). Supplement with 25 % (v/v) of heat-inactivated fetal bovine serum (Hyclone).
-
7.
2 % Avertin anesthetizing solution: 2.4 g 2,2,2-Tribromoethanol (99 %) (Alfa Aesar), 1.488 ml Tert-amyl alcohol (Sigma), 118.5 ml 1× PBS.
-
8.
1 cc Insulin syringe (28G ½).
-
9.
Gas mixture: 5 % CO2, 5 % O2, and 90 % N2.
-
10.
Cell culture flask (150 cm2 with plug seal).
-
11.
Biosafety laminar flow hood.
-
12.
Incubator.
-
13.
Orbital shaker.
-
14.
Phase-contrast microscope.
-
15.
Immersion oil.
-
16.
Forceps.
-
17.
Scissors.
-
18.
70 % Ethanol.
-
19.
7 in. glass Pasteur pipette.
-
20.
Pipette bulb.
-
21.
50 ml Polypropylene tubes.
-
22.
1.5 ml Microcentrifuge tubes.
-
23.
200 μl and 20 μl micropipettes with corresponding plastic tips.
-
24.
10 ml Serological pipettes (sterile).
-
25.
Pipette aid.
-
26.
Water bath set at 37 °C.
-
27.
Swing bucket table-top centrifuge with no-brake capacity.
2.1.3 Schizont Purification
-
1.
Histodenz stock solution: 138 g of Histodenz (Sigma) in 500 ml of stock buffer. Autoclave immediately at 120 °C for 20 min and store at 4 °C.
-
2.
Stock buffer medium: 5 mM Tris/HCl, 3 mM KCl, 0.3 mM CaNa2EDTA; pH 7.5.
-
3.
1× PBS.
-
4.
Swing bucket table-top centrifuge with no-brake capacity.
-
5.
7 in. glass Pasteur pipette.
-
6.
Pipette bulb.
2.1.4 Schizont Electroporation and Injection
-
1.
Basic Parasite Nucleofector Kit 2: Basic Parasite Nucleofector Solution 2, Supplement P2, cuvettes, pipettes (Lonza).
-
2.
Nucleofector II Electroporator (Amaxa).
-
3.
Purified schizonts as described in Subheading 2.1.3.
-
4.
Linearized transfection vector (5–10 μg in 10 μl of PCR-grade water for each transfection).
-
5.
1.5 ml Microcentrifuge tubes.
-
6.
200 μl Micropipette with corresponding plastic tips.
-
7.
1 cc Insulin syringe (28G ½).
-
8.
Infrared heating lamp.
-
9.
Swiss Webster mice (5–8 weeks old; ~25 g; considering one mouse per each transfection, usually five mice are needed for a transfection experiment).
2.1.5 Selection of Transfected Parasites by Treatment with Pyrimethamine in Drinking Water
-
1.
Pyrimethamine solution: Pyrimethamine powder (Sigma) dissolved in DMSO (Sigma) to a final concentration of 7 mg/ml and diluted 100× with tap water. pH must be adjusted to 3.5–5 using 1 M HCl. Protect from light.
-
2.
Opaque drinking bottles (if opaque drinking bottles are not available, cover the bottles in foil to protect the pyrimethamine from light).
-
3.
Glass slides (25 × 75 mm).
-
4.
Methanol.
-
5.
Giemsa stain (Sigma).
2.1.6 Cryopreservation of Drug-Resistant Parasites
-
1.
Cryovials.
-
2.
Scissors.
-
3.
Forceps.
-
4.
7 in. glass Pasteur pipette.
-
5.
Pipette bulb.
-
6.
2 % Avertin anesthetizing solution: 2.4 g 2,2,2-Tribromoethanol (99 %) (Alfa Aesar), 1.488 ml Tert-amyl alcohol (Sigma), 118.5 ml 1× PBS.
-
7.
1 cc Insulin syringe (28G ½).
-
8.
Heparin solution (200 U/ml in 1× PBS).
-
9.
Parasite freezing solution: 10 % (v/v) Glycerol/Alsever’s Solution (Sigma).
-
10.
200 μl Micropipette with corresponding plastic tips.
-
11.
1.5 ml Microcentrifuge tubes.
2.2 Evaluation of the Biological Characteristics of Transgenic Parasites
2.2.1 Exflagellation of Male Gametocytes
-
1.
Mouse infected with P. berghei strain under evaluation (see Note 2 ).
-
2.
Heparin solution (40 U/ml).
-
3.
Complete ookinete medium: RPMI 1640 medium with l-glutamine (Gibco), 20 % (v/v) heat-inactivated fetal bovine serum (FBS) (Hyclone), 25 mM Hepes buffer, 2 mM glutamine, 367 μM hypoxanthine, 23.8 mM sodium bicarbonate, 5 U/ml penicillin, 5 mg/ml streptomycin, 100 μM xanthurenic acid. Adjust pH to 7.4.
-
4.
1.5 ml Microcentrifuge tubes.
-
5.
Scissors.
-
6.
Methanol.
-
7.
Giemsa stain (Sigma).
-
8.
100× Giemsa buffer: 59.24 g Na2HPO4, 36.38 g NaH2PO4H2O, 1000 ml deionized water.
-
9.
10 μl Micropipette with corresponding plastic tips.
-
10.
Glass slides (25 × 75 mm).
-
11.
Microscope cover slip (18 × 18 mm).
-
12.
Mineral oil.
-
13.
Phase-contrast microscope.
2.2.2 Assessment of Midgut Oocyst Development
-
1.
Female Anopheles stephensi mosquitoes: 10–14 days after infectious blood meal (see Note 3 ).
-
2.
Aspirator for collecting mosquitoes.
-
3.
Ice bucket/ice.
-
4.
70 % Ethanol.
-
5.
1× PBS.
-
6.
Mercury dibromofluorescein solution: 0.1 % (w/v) Mercury dibromofluorescein disodium salt (Sigma) in 1× PBS.
-
7.
Two plastic Petri dishes.
-
8.
Two pairs of fine-point forceps.
-
9.
One-side concavity glass slide (Thomas Scientific).
-
10.
Glass slides (25 × 75 mm).
-
11.
Microscope cover slip (22 × 60 mm).
-
12.
Dissecting stereoscopic microscope.
-
13.
Phase-contrast microscope.
-
14.
Laboratory counter.
2.2.3 Salivary Gland Infection Rate and Mosquito Sporozoite Yield
-
1.
Female A. stephensi mosquitoes: 18–22 days after infectious blood meal (see Note 3 ).
-
2.
Aspirator for collecting mosquitoes.
-
3.
Ice bucket/ice.
-
4.
70 % Ethanol.
-
5.
1× PBS.
-
6.
1× PBS with 1 % (w/v) bovine serum albumin (BSA) (Sigma).
-
7.
Hanks’ Balanced Salt Solution (HBSS) (Gibco) supplemented with 1 % heat-inactivated mouse serum (Sigma).
-
8.
200 μl Micropipette with corresponding plastic tips.
-
9.
10 μl Micropipette with corresponding plastic tips.
-
10.
Two plastic Petri dishes.
-
11.
One pair of fine-point forceps.
-
12.
1 cc Insulin syringe (28G ½)
-
13.
Glass slides (25 × 75 mm).
-
14.
Microscope cover slip (22 × 60 mm).
-
15.
7 in. glass pipette.
-
16.
Pipette bulb.
-
17.
Benchtop microcentrifuge (set at 4 °C).
-
18.
Cell strainer (70 μm—nylon).
-
19.
1.5 ml Microcentrifuge tubes.
-
20.
Pestle to fit microcentrifuge tubes.
-
21.
Hemocytometer.
-
22.
Dissecting stereoscopic microscope.
-
23.
Phase-contrast microscope.
-
24.
Laboratory counter.
-
25.
Paper towels.
2.2.4 Development of Blood-Stage Forms upon Infectious Mosquito Bites
-
1.
Female A. stephensi mosquitoes: 18–22 days after infectious blood meal (see Note 3 ).
-
2.
Mice (n = 5–10): 5–8-week-old C57BL/6 mice .
-
3.
2 % Avertin anesthetizing solution: 2.4 g 2,2,2-Tribromoethanol (99 %) (Alfa Aesar), 1.488 ml Tert-amyl alcohol (Sigma), 118.5 ml 1× PBS.
-
4.
Same materials as for salivary gland infection rate and mosquito sporozoite yield.
-
5.
12 oz. paper cups individually capped with mosquito netting (polyester mesh).
-
6.
Methanol.
-
7.
Giemsa stain (Sigma).
-
8.
100× Giemsa buffer: 59.24 g Na2HPO4, 36.38 g NaH2PO4H2O, 1000 ml deionized water.
-
9.
Timer/stop watch.
-
10.
Scissors.
-
11.
Mineral oil.
-
12.
CO2 chamber for euthanizing mice.
-
13.
Mouse ear tags with corresponding applicator.
3 Methods
3.1 Transfection Plasmid Design
The careful design of transfection plasmids is critical for the development of transgenic rodent malaria parasites. We describe a few factors that should be considered when designing a transfection vector:
-
Length of the homology arms flanking a replacement construct—Longer homology arms increase the frequency and efficiency of double-homologous recombination events [15]. Based on our experience, we recommend homology arms 800–1000 base pairs in length.
-
Position of the drug-selectable markers—When using a drug-selectable marker it is necessary to account for its position (upstream/downstream) in relation to the gene or target of interest. This is because, in some cases, the location of the drug-selectable marker in the replacement construct can interfere with regulatory sequences of genes that are in close proximity to the gene of interest and are critical for parasite development.
-
Length of the untranslated regions (UTRs)—We recommend that transfection constructs should incorporate a minimum of 500 base pairs of UTRs upstream and downstream of the gene(s) of interest and the drug-selectable marker. UTRs encode regulatory sequences recognized by proteins needed for gene transcription.
-
Codon harmonization—It is advisable to codon harmonize the replacement construct in order to optimize protein synthesis and folding in the transgene host [17]. In addition, significant differences in codon usage between different Plasmodium species have been reported [18].
3.2 Transfection of Rodent Malaria Parasites
The guidelines and methodology provided below have been previously described by Philip et al. [15] and Janse et al. [13]. We encourage readers to review the original methodology for additional details.
3.2.1 Establishment of Infection and Subpassage to Schizont Donor Mice
-
1.
On day 0, infect a mouse with a wild-type P. berghei blood aliquot by intraperitoneal (I.P.) injection
-
2.
2–3 days later, perform a blood smear on a glass slide.
-
3.
Fix the smear with methanol for 30 s.
-
4.
Without rinsing the slide, stain the smear for 15 min using a 10 % solution of Giemsa stain diluted in 1× Giemsa buffer.
-
5.
Observe the slide under the phase-contrast microscope (100×, using immersion oil).
-
6.
If parasitemia has reached 1–3 %, draw a blood sample by snipping the tip of the tail—no more than 3 mm from the end and pipette 10–15 μl of blood into a microcentrifuge tube containing 500 μl of HBSS-1 % mouse serum. Mix the sample thoroughly.
-
7.
Inject (I.P.) 200 μl of the blood sample prepared in step 6 into two mice.
3.2.2 Schizont In Vitro Culture
-
1.
3–4 days after infection, check the blood-stage parasitemia of the schizont donor mice by performing a thin blood smear. If parasitemia is 1–3 % proceed to next step; otherwise, wait another day and check again at the same time of day (see Note 4 ).
-
2.
If proceeding with the experiment, gather all needed materials inside a biosafety laminar flood hood; make sure to set up the orbital shaker inside the incubator; place ~50 μl of heparin solution in a 50 ml polypropylene tube; and warm up the complete culture medium by placing the container in a water bath set at 37 °C.
-
3.
Anesthetize mice using 400–500 μl of 2 % Avertin, delivered by I.P. injection (see Note 1 ).
-
4.
Carefully, working one mouse at a time, moisten the torso and abdomen with 70 % ethanol and place the mouse inside of the hood.
-
5.
Collect 1–1.5 ml of blood in the polypropylene tube with heparin. With a mouse firmly restrained, perform a small incision on the right side of the torso. Collect the blood flowing through the right axillary vein using the 7 in. Pasteur pipette attached to the bulb. Efforts should be made to work swiftly and to minimize discomfort to animals; after blood collection euthanize animal according to your institute’s protocol (see Note 1 ).
-
6.
Once blood collection is completed (estimate ~2 ml from two mice), add 20 ml of pre-warmed culture medium using the serological pipette and homogenize with the blood by gentle inversion.
-
7.
Centrifuge the sample at 200 × g for 8 min at room temperature in a swing bucket table-top centrifuge without brake. In the meantime, place 50 ml of warm culture medium in the cell culture flask.
-
8.
Using a serological pipette, gently remove the supernatant trying not to disturb the red blood cell pellet.
-
9.
Add 30 ml to the red blood cells and mix by gentle inversion.
-
10.
Carefully decant the contents of the tube into the cell culture flask previously filled with culture medium warmed to 37 °C.
-
11.
Wash the 50 ml tube by adding 20 ml of culture medium and mixing by inversion. Then, add this to the culture flask.
-
12.
Gas the culture with the 5 % CO2, 5 % O2, and 90 % N2 gas mixture (this usually takes 1 min). Cap the culture flask and adjust the plug seal cap tightly.
-
13.
Place the flask in the incubator set at 37 °C and rest it for 5 min.
-
14.
Start the orbital shaker at minimum speed (20–30 rpm), enough to keep the cells in suspension.
-
15.
Incubate the parasite culture overnight (~18 h).
-
16.
Take a 200–500 μl aliquot from the culture and place it in a microcentrifuge tube.
-
17.
Pellet the red blood cells by spinning the sample at maximum speed (16,000 × g) for 5–10 s and gently aspirate the supernatant.
-
18.
Take 10–20 μl of the red blood cell pellet and make a thin blood smear. Giemsa stain the slide and examine under the phase-contrast microscope. If 70–80 % of parasites are healthy looking, proceed to the next step (see Note 5 ).
3.2.3 Schizont Purification
-
1.
Bring the Histodenz solution to room temperature. Then, prepare 50 ml of a 55 % Histodenz/PBS solution (v/v).
-
2.
Transfer the schizont culture to three 50 ml polypropylene tubes (~30 ml per tube).
-
3.
Using a serological pipette attached to a pipette aid, gently add 15 ml of the Histodenz solution to each tube. Make sure to take the pipette to the bottom of the tube, under the culture suspension, before gradually releasing the Histodenz solution in order to prevent disruption of the gradient. If performed correctly, a contrasting division is seen between the two suspensions.
-
4.
Centrifuge for 20 min at 450 × g in a swing bucket table-top centrifuge, without brake and gentle acceleration (as an example, set acceleration to “3” in Eppendorf centrifuge model 5810R).
-
5.
Using a 7 in. Pasteur pipette attached to a bulb, gently collect the brown-colored layer at the interface between the two suspensions, and place this material in a clean 50 ml polypropylene tube. Typically, ~30 ml is collected from three tubes.
-
6.
Keep the gradient tubes as the culture medium from the top layers is needed for subsequent steps.
-
7.
To wash and concentrate the parasites, add 20 ml of culture medium from the previous step to the tube containing the schizonts and centrifuge at 200 × g for 8 min.
-
8.
Discard the supernatant and resuspend the pellet in 5 ml of culture medium from step 6.
3.2.4 Schizont Electroporation and Injection
-
1.
Start warming up mice by placing them at a safe distance under the infrared heating lamp. Monitor animals regularly for any signs of discomfort.
-
2.
Distribute the schizont-containing suspension in five microcentrifuge tubes (1 ml in each tube). One tube is used for each transfection.
-
3.
Prepare Nucleofector solutions and DNA material. For each transfection mix 90 μl of Basic Parasite Nucleofector Solution 2, 20 μl of Supplement P2, and 5–10 μl of linearized plasmid DNA (1 μg/μl).
-
4.
Working one tube at a time, pellet the cells by centrifugation (5 s at maximum speed, 16,000 × g) and discard the supernatant.
-
5.
Resuspend the schizonts in 100 μl of the previously made transfection mix.
-
6.
Using a micropipette, transfer the parasite-containing mixture to a cuvette. Avoid introducing bubbles when dispensing the solution.
-
7.
Transfect using the Amaxa Nucleofector set to protocol U-033 to electroporate.
-
8.
Immediately after transfection, add 50 μl of culture medium (from step 6 of Subheading 3.2.3) to the cuvette.
-
9.
Transfer the contents of the cuvette to a microcentrifuge tube using one of the plastic pipettes provided by the transfection kit.
-
10.
Quickly inject the transfected parasites into the tail vein of a mouse.
-
11.
Repeat steps 4–10 until all parasite material is used.
-
12.
24 h after injection of transfected parasites, perform a thin blood smear to determine if parasites survived the transfection procedures.
3.2.5 Selection of Transfected Parasites by Treatment with Pyrimethamine in Drinking Water
Currently, the most commonly used drug-selectable marker for transfection of P. berghei parasites is the dihydrofolate reductase-thymidylate synthase (DHFR-TS), an enzyme involved in folate metabolism. The DHFR-TS genes of P. berghei and Toxoplasma gondii , as well as the human DHFR gene, are capable of conferring resistance to pyrimethamine when administered in drinking water [19, 20].
-
1.
Upon confirmation of blood-stage parasitemia, provide mice pyrimethamine in drinking water.
-
2.
Drug treatment takes place for 4–7 days.
-
3.
7 days after transfection, determine the presence of blood-stage parasites by Giemsa-stained blood smears.
-
4.
Once parasitemia has reached 1–5 %, cryopreserve two to three blood aliquots from each mouse and use the remaining material for DNA analysis.
3.2.6 Cryopreservation of Drug-Resistant Parasites
-
1.
Anesthetize mice using 400–500 μl of 2 % Avertin, delivered by I.P. injection.
-
2.
Collect blood from each mouse as described in Subheading 3.2.2 (step 5).
-
3.
Place the collected blood (~1 ml/mouse) in a microcentrifuge tube containing 50 μl of heparin (200 U/ml) and mix thoroughly.
-
4.
Distribute 100 μl of blood in each cryovial and mix it with 200 μl of parasite-freezing solution.
-
5.
Place the cryovials in an isopropanol cryobox and store them at −80 °C. Samples can be then frozen directly in liquid nitrogen.
-
6.
Use the remaining blood for DNA extraction and molecular analysis.
3.3 Evaluation of the Biological Characteristics of Transgenic Parasites
It is critical to ensure that transgenic parasites remain as infectious as their unmodified counterparts, i.e., the parental strain. The assays described below should allow a thorough assessment of their developmental characteristics.
3.3.1 Exflagellation of Male Gametocytes
-
1.
To determine the presence of blood-stage gametocytes, perform a blood smear from the infected mouse by snipping the tip of the tail, no more than 3 mm from the end, and place a drop of blood on a glass slide.
-
2.
Using another glass slide, make a thin smear of the blood drop.
-
3.
Fix the smear with methanol for 30 s.
-
4.
Without rinsing the slide, stain the smear for 15 min using a 10 % solution of Giemsa stain diluted in 1× Giemsa buffer.
-
5.
Determine the presence of gametocytes. Visualize several fields using the 100× objective of the phase-contrast microscope with mineral oil.
-
6.
If gametocytes are observed (ideally, one gametocyte per field), combine in a 1.5 ml microcentrifuge tube 7 μl of complete ookinete medium, 1.5 μl of heparin, and 1.5 μl of mouse blood.
-
7.
Mix well and incubate for 15–20 min at room temperature.
-
8.
Place 10 μl of this mix on top of a glass slide. Then, place a glass cover slip on top of the drop, making sure that the blood distributes evenly under the cover slip.
-
9.
Observe the slide with a phase-contrast microscope, first using the 40× objective and then switching to the 100× (using mineral oil). Look for exflagellating male gametocytes, characterized by highly motile forms erupting from the infected red blood cells.
3.3.2 Assessment of Midgut Oocyst Development
-
1.
Aspirate 15–25 mosquitoes and place them in a Petri dish containing 10 ml of 70 % ethanol (mosquitoes will die upon contact with the ethanol).
-
2.
Using fine-point forceps, transfer the mosquitoes to a Petri dish with 5 ml of 1× PBS.
-
3.
Working under the stereoscopic microscope, gently hold the mosquitoes by the thorax and carefully pull out the midguts through the rear end of the abdomen (see Note 6 ).
-
4.
Using fine-point forceps, collect the midguts in a one-side concavity containing 200 μl of 1× PBS.
-
5.
Transfer the midguts to a one-side concavity slide containing 200 μl of mercury dibromofluorescein solution and incubate at room temperature for 20 min.
-
6.
Rinse the midguts by transferring them to a one-side concavity slide containing 200 μl of 1× PBS; incubate at room temperature for 10 min.
-
7.
Using the fine-point forceps, transfer the midguts to a glass slide and arrange them in a way that facilitates microscope observation. Use a few microliters of 1× PBS to keep them wet and place a cover slip on top of the slide.
-
8.
Observe the slide with a phase-contrast microscope, first using the 10× objective and then switching to the 40×.
-
9.
Count the number of oocysts in each mosquito midgut. P. berghei-infected midguts in our laboratory-adapted wild-type strain tend to harbor 50–100 oocysts each. However, some mosquitoes might develop significantly milder infections.
3.3.3 Salivary Gland Infection Rate and Mosquito Sporozoite Yield
The efficient migration of sporozoites to mosquito salivary glands is critical for evaluating the biology and immunogenicity of transgenic parasites in the mammalian host.
3.3.3.1 Salivary Gland Isolation and Infection Assessment
-
1.
Aspirate 15–25 mosquitoes and place them in a Petri dish containing 10 ml of 70 % ethanol (mosquitoes will die upon contact with the ethanol).
-
2.
Using fine-point forceps, rinse the mosquitoes by placing them in a Petri dish with 5 ml of 1× PBS.
-
3.
Place the mosquitoes on a paper towel on ice during the isolation of the salivary glands.
-
4.
Place a glass slide under the stereoscopic microscope and wet the slide’s surface with 100–150 μl of cold 1× PBS-1 % BSA.
-
5.
Working in groups of five to ten mosquitoes, isolate the salivary glands on the glass slide. Use the fine-point forceps to gently hold each mosquito by its thorax and carefully pull the head away from the rest of the body using the needle of a 1 cc insulin syringe. If performed correctly, the trilobed salivary glands should remain attached to the mosquito’s head.
-
6.
Manipulate the mosquito’s head to remove the salivary glands using the needle of a 1 cc insulin syringe. Always keep the glands wet and separated from the mosquito debris.
-
7.
Using a paper towel, carefully clean the glass slide to remove all the mosquito material. Avoid disturbing the salivary glands.
-
8.
Add a drop of 1× PBS-1 % BSA to wet the surface of the glass slide. Use the 1 cc insulin syringe to evenly distribute the salivary glands, and try to keep them 0.5–1 cm away from each other.
-
9.
Place a cover slip on top of the salivary glands and focus on them using a phase-contrast microscope with a 40× objective. With the tip of a pencil, gently squeeze the gland under the objective. Infected glands usually harbor hundreds of sporozoites that can be identified by their crescent shape.
-
10.
Determine the proportion of infected salivary glands to calculate the mosquito infection rate.
3.3.3.2 Sporozoite Purification and Mosquito Sporozoite Yield
-
1.
Isolate the mosquito salivary glands as described in Subheading “Salivary Gland Isolation and Infection Assessment” (steps 1–6) (see Note 7 ).
-
2.
Count the number of dissected mosquitoes before disposing of the carcasses. It may be helpful to collect the carcasses in groups of three to five mosquitoes using fine-point forceps, place them over a damp paper towel, and then count. Write down the number of dissected mosquitoes.
-
3.
Carefully aspirate the salivary glands using the 7 in. glass pipette attached to the bulb and place them in a previously chilled microcentrifuge tube containing 50 μl of HBSS-1 % mouse sera. Keep the glands wet at all times and try to keep the overall collection volume under 500 μl.
-
4.
Spin the glands for 3 min at 3300 × g in a microcentrifuge to facilitate homogenization.
-
5.
Disrupt the glands to release the sporozoites by squeezing and turning the pestle against the bottom of the tube. Repeat this process until no large pieces of mosquito debris can be seen.
-
6.
Spin the microcentrifuge tube at 600 × g for 3 min. Aspirate all the supernatant and pass it through a cell strainer into a clean microcentrifuge tube sitting on ice. This removes any large pieces of mosquito debris that could still be present in the salivary gland homogenate.
-
7.
Repeat steps 5 and 6 using 150 μl of HBSS-1 % mouse sera and combine with the previously collected material. At the end of this process, determine the total volume of the sporozoite suspension.
-
8.
Thoroughly mix the sporozoite suspension and place 10 μl on the hemocytometer. Let the sporozoites settle for 5–10 min at room temperature before counting. Allowing the parasites to get to the bottom of the hemocytometer significantly facilitates counting.
-
9.
Use a phase-contrast microscope (40× objective) to determine the number of sporozoites by counting the four corner sections of the hemocytometer (each subdivided in 16 squares in a 4 × 4 arrangement). The parasites look like black eye lashes and are approximately 10 μm in length.
-
10.
Determine the mosquito sporozoite yield by performing the following calculations: [(number of counted sporozoites in the four corner sections)/4] × 10 = number of sporozoites/μl. Then, multiply this number by the total volume of the sporozoite suspension to obtain the total amount of sporozoites. Finally, the number of sporozoites per mosquito is total amount of sporozoites/number of mosquitoes dissected.
3.3.4 Development of Blood-Stage Forms upon Infectious Mosquito Bites
This is an assessment of the overall infective capacity of a parasite strain. We evaluate the parasite’s capacity to infect and develop within hepatocytes as well as the subsequent initiation of blood infection. Most important, exposure to infectious mosquito bites is the preferred method to evaluate the efficacy of different vaccine formulations as this is the natural route of infection.
-
1.
Determine the salivary gland infection rate as detailed in Subheading “Salivary Gland Isolation and Infection Assessment.”
-
2.
Based on the previous estimate, sort the needed mosquitoes in each of the paper cups (see Note 8 ). Label each cup to match the ear tag numbers of the mice meant for this experiment and starve the mosquitoes for 12–24 h.
-
3.
Tag mice.
-
4.
The day of the experiment, anesthetize mice using 2 % Avertin (200–250 μl for a ~20 g mouse).
-
5.
Place each mouse on top of its corresponding paper cup containing the starved mosquitoes. Cover the cups using a piece of cloth or towel.
-
6.
Allow the mosquitoes to feed on the mice for 5–10 min. Then, return mice to their cages and monitor their recovery.
-
7.
Working one cup at a time, aspirate the mosquitoes, place them on a Petri dish with 5 ml of 70 % ethanol, and determine how many fed on each mouse. This can be easily done by looking at their abdomens for the presence of blood (it may be helpful to perform observations using the stereoscopic microscope).
-
8.
Using a Petri dish with 5 ml of 1× PBS, rinse the mosquitoes that blood-fed in each cup and determine the salivary gland infection rate of the blood-fed mosquitoes (Subheading “Salivary Gland Isolation and Infection Assessment”). At this stage, score the dissected salivary glands of the blood-fed mosquitoes as “uninfected” or “infected” and do not focus on the absolute number of sporozoites obtained from each mosquito. These values can be used to determine how many infectious blood meals each mouse received.
-
9.
To determine the onset of patency, start performing daily thin blood smears 4 days after the mosquito feeding assay. For accuracy, perform blood smears at the same time each day (i.e., exactly at 96, 120, and 144 hours after the assay).
-
10.
Giemsa-stain the blood smears by fixing them with methanol for 30 s and then staining for 15 min with Giemsa working solution (10 % Giemsa stain in 1× Giemsa buffer). Rinse the slides with tap water and dry at room temperature.
-
11.
Read the slides using the phase-contrast microscope (100× objective) with mineral oil.
-
12.
Once the presence of blood-stage parasites becomes evident, euthanize mice immediately in a CO2 chamber or according to your animal study protocol. In our experience, a naïve mouse that received three infectious mosquito bites will become patent for blood-stage parasites 4–6 days post-infection.
-
13.
Continue performing daily blood smears on mice that do not develop blood-stage parasitemia for up to 14 days after the mosquito biting assay. After this time period, it is very unlikely that they will develop blood stages.
-
14.
With the collected data, estimate the percentage of mice that developed blood-stage parasitemia upon infected mosquito bites and the pre-patent period for the tested parasite line. Pre-patent period = (1st day of positive blood smear for mouse 1 + 1st day of positive blood smear for mouse 2 + 1st day of positive blood smear for mouse 3, etc.)/(total number of mice positive for blood stage parasitemia).
4 Notes
-
1.
All experiments involving the use of mice should be approved by the relevant ethics committees and strictly adhere to local and international regulations. Appropriate training is necessary for all personnel handling animals.
-
2.
To procure optimal blood-stage gametocytemia when starting from a frozen blood sample, infection must be first established in a starter mouse and then subpassaged to additional naïve mice. Infect a mouse with a frozen blood aliquot and allow parasitemia to reach 3 %. Draw a blood sample from this mouse (~50–100 μl), dilute it to 1 % in 1× PBS, and infect a mouse I.P. with 200 μl. 2–3 days later, proceed to the exflagellation assay.
-
3.
Infection of 3–7-day-old female A. stephensi mosquitoes is performed with P. berghei-infected mice in which the presence of exflagellating gametocytes has been verified. Mosquitoes are allowed to feed on anesthetized mice for 30 min (use 200–250 μl of 2 % Avertin for an ~20 g mouse). Knock down the mosquitos by placing them at 4 °C for 5–10 min. Then, working in a cold room, dispose of the mosquitoes that did not take a blood meal (i.e., do not have blood in their abdomens) and keep those ones that did. Mosquitoes are kept at 19 °C until P. berghei sporozoites develop and reach the salivary glands (18–22 days after the infectious blood meal).
-
4.
Parasitemia above 3 % is not recommended and could impair the development of schizonts in culture. Higher parasitemia will result in multiply infected erythrocytes or in parasites residing in normocytes (older erythrocytes) and not in reticulocytes [13].
-
5.
Healthy, viable schizonts are characterized by having 12–16 merozoites within one erythrocyte and a cluster of hemozoin. However, the red cell membrane is occasionally broken when blood is thinly smeared, resulting in merozoite release. Deteriorated schizonts tend to display a compact morphology, making it difficult to distinguish individual merozoites. If at this stage schizonts look underdeveloped, then gas the culture again and incubate for an additional 2–3 h before starting the purification procedure.
-
6.
When isolating midguts, it is important to properly distinguish them from other structures of the mosquito anatomy. It is not unusual for inexperienced researchers to confuse the midguts with structures belonging to the hindgut or even the reproductive system. A practical way to distinguish the midguts is by looking for the Malpighian tubes, slender threadlike structures attached to the base of the midgut.
-
7.
In our experience, it is preferable to use HBSS-1 % mouse serum instead of 1× PBS-1 % BSA if the isolated sporozoites are going to be injected into mice.
-
8.
When testing a newly developed transgenic line, it is useful to determine the minimum number of infected mosquitoes that must take a blood meal for mice to develop blood-stage parasitemia. Sporozoites from both our wild-type and transgenic parasite lines can infect mice and result in blood-stage parasitemia 4–5 days after feeding of one to three infected mosquitoes.
References
Zavala F, Tam JP, Barr PJ et al (1987) Synthetic peptide vaccine confers protection against murine malaria. J Exp Med 166:1591–1596
Kester KE, Cummings JF, Ofori-Anyinam O et al (2009) Randomized, double-blind, phase 2a trial of falciparum malaria vaccines RTS, S/AS01B and RTS, S/AS02A in malaria-naive adults: safety, efficacy, and immunologic associates of protection. J Infect Dis 200:337–346
Agnandji ST, Lell B, Soulanoudjingar SS et al (2011) First results of phase 3 trial of RTS, S/AS01 malaria vaccine in African children. N Engl J Med 365:1863–1875
Agnandji ST, Lell B, Fernandes JF et al (2012) A phase 3 trial of RTS, S/AS01 malaria vaccine in African infants. N Engl J Med 367:2284–2295
The RTS SCTP (2014) Efficacy and safety of the RTS, S/AS01 malaria vaccine during 18 months after vaccination: a phase 3 randomized, controlled trial in children and young infants at 11 African sites. PLoS Med 11(7), e1001685
Doolan DL, Southwood S, Freilich DA et al (2003) Identification of Plasmodium falciparum antigens by antigenic analysis of genomic and proteomic data. Proc Natl Acad Sci U S A 100:9952–9957
Heppner DG Jr, Kester KE, Ockenhouse CF et al (2005) Towards an RTS, S-based, multi-stage, multi-antigen vaccine against falciparum malaria: progress at the Walter Reed Army Institute of Research. Vaccine 23:2243–2250
Cockburn I (2013) Chimeric parasites as tools to study Plasmodium immunology and assess malaria vaccines. Methods Mol Biol 923:465–479
Persson C, Oliveira GA, Sultan AA et al (2002) Cutting edge: a new tool to evaluate human pre-erythrocytic malaria vaccines: rodent parasites bearing a hybrid Plasmodium falciparum circumsporozoite protein. J Immunol 169(12):6681–6685
Tewari R, Spaccapelo R, Bistoni F et al (2002) Function of region I and II adhesive motifs of Plasmodium falciparum circumsporozoite protein in sporozoite motility and infectivity. J Biol Chem 277(49):47613–47618
Espinosa DA, Yadava A, Angov E et al (2013) Development of a chimeric Plasmodium berghei strain expressing the repeat region of the P. vivax circumsporozoite protein for in vivo evaluation of vaccine efficacy. Infect Immun 81:2882–2887
Bauza K, Malinauskas T, Pfander C et al (2014) Efficacy of a Plasmodium vivax malaria vaccine using ChAd63 and modified vaccinia Ankara expressing thrombospondin-related anonymous protein as assessed with transgenic Plasmodium berghei parasites. Infect Immun 82:1277–1286
Janse CJ, Ramesar J, Waters AP (2006) High-efficiency transfection and drug selection of genetically transformed blood stages of the rodent malaria parasite Plasmodium berghei. Nat Protoc 1(1):346–356
Janse CJ, Franke-Fayard B, Mair GR et al (2006) High efficiency transfection of Plasmodium berghei facilitates novel selection procedures. Mol Biochem Parasitol 145:60–70
Philip N, Orr R, Waters AP (2013) Transfection of rodent malaria parasites. Methods Mol Biol 923:99–125
Wengelnik K, Spaccapelo R, Naitza S et al (1999) The A-domain and the thrombospondin-related motif of Plasmodium falciparum TRAP are implicated in the invasion process of mosquito salivary glands. EMBO J 18:5195–5204
Angov E, Hillier CJ, Kincaid RL et al (2008) Heterologous protein expression is enhanced by harmonizing the codon usage frequencies of the target gene with those of the expression host. PLoS One 3(5)
Yadav MK, Swati D (2012) Comparative genome analysis of six malarial parasites using codon usage bias based tools. Bioinformation 8:1230–1239
van Dijk MR, McConkey GA, Vinkenoog R et al (1994) Mechanisms of pyrimethamine resistance in two different strains of Plasmodium berghei. Mol Biochem Parasitol 68:167–171
Fidock DA, Wellems TE (1997) Transformation with human dihydrofolate reductase renders malaria parasites insensitive to WR99210 but does not affect the intrinsic activity of proguanil. Proc Natl Acad Sci U S A 94:10931–10936
Acknowledgements
Research in Dr. Fidel Zavala’s laboratory is supported by PATH Malaria Vaccine Initiative (MVI) and the National Institutes of Health (NIH) (grant number AI44375). D.A.E. received a predoctoral fellowship from the Johns Hopkins Malaria Research Institute. The authors thank the Bloomberg Family Foundation for continued support. This research was supported in part by the Intramural Research Program of NIAID, NIH; A.J.R. is supported by the Intramural Research Program of NIAID, NIH.
Author information
Authors and Affiliations
Corresponding author
Editor information
Editors and Affiliations
Rights and permissions
Copyright information
© 2016 Springer Science+Business Media New York
About this protocol
Cite this protocol
Espinosa, D.A., Radtke, A.J., Zavala, F. (2016). Development and Assessment of Transgenic Rodent Parasites for the Preclinical Evaluation of Malaria Vaccines. In: Thomas, S. (eds) Vaccine Design. Methods in Molecular Biology, vol 1403. Humana Press, New York, NY. https://doi.org/10.1007/978-1-4939-3387-7_33
Download citation
DOI: https://doi.org/10.1007/978-1-4939-3387-7_33
Published:
Publisher Name: Humana Press, New York, NY
Print ISBN: 978-1-4939-3385-3
Online ISBN: 978-1-4939-3387-7
eBook Packages: Springer Protocols